Malaria: Combating a Global Health Challenge
Introduction
Malaria. Just the word alone might conjure up images of tropical landscapes, mosquito bites, and crowded clinics. But what exactly is malaria, and why is it still such a major global health challenge in 2025?
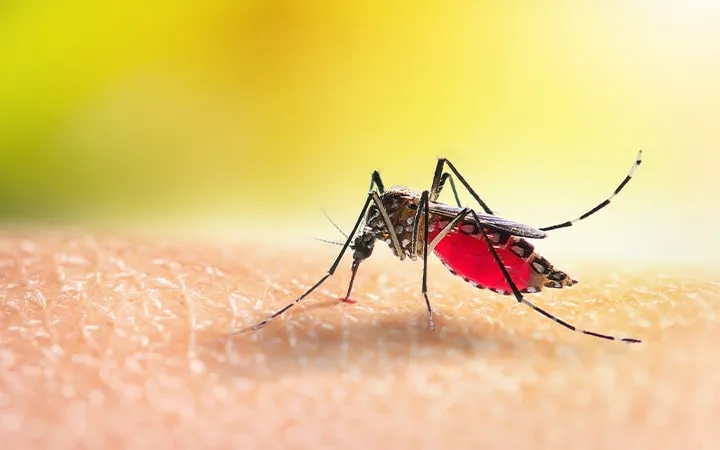
Malaria is a life-threatening disease caused by parasites of the genus Plasmodium, transmitted to people through the bites of infected female Anopheles mosquitoes. It’s both ancient and persistent—an illness that has plagued humanity for thousands of years and continues to claim hundreds of thousands of lives annually. According to the World Health Organization, in recent years, over 240 million cases of malaria have been reported globally each year. That’s nearly the entire population of Indonesia falling ill—every single year.
It’s worth noting, though, that malaria is a shapeshifter. The parasite has evolved clever ways to hide from the immune system. The mosquitoes that carry it are equally crafty, adapting to insecticides and shifting their feeding patterns. Add to that a tangle of socioeconomic factors—poverty, weak health systems, conflict zones, climate variability—and you get a disease that’s hard to pin down and even harder to wipe out.
The fight against malaria has a long and storied history. From the use of quinine in the 17th century to the mass distribution of insecticide-treated nets in the 21st, there have been waves of progress. In the mid-20th century, the World Health Organization launched the Global Malaria Eradication Program—a bold and partially successful effort that wiped out malaria in large parts of Europe, North America, and parts of Asia. However, that campaign ultimately stalled in sub-Saharan Africa, where malaria is most entrenched.
Fast forward to today, and we’re at a fascinating crossroads. The past two decades have seen renewed commitment, fresh funding, and groundbreaking science. New vaccines have emerged. Gene-editing tools are being explored to make mosquitoes incapable of carrying the parasite. Even AI and data science are playing a role in predicting outbreaks and optimizing responses.
So again, why does malaria still matter in 2025?
Because despite the progress, malaria still kills over 600,000 people every year—most of them children under the age of five. Because a single missed diagnosis or failed treatment can mean death. And because with the right mix of strategy, science, and global cooperation, malaria might actually be beatable within our lifetime.
Malaria, like many persistent global threats, reminds us that infectious diseases don’t always need a breaking-news moment to matter. They simmer in the background, evolve quietly, and resurface when systems falter. This slow, insidious pattern echoes the kind of emerging risk that’s led experts to flag Disease X—a hypothetical but entirely plausible next pandemic threat that shares many of the uncertainties malaria once posed.
This chapter will dive deeper into what causes malaria, how it spreads, how it affects the body, how we treat and prevent it, and what’s next on the horizon. But for now, let’s take a closer look at the parasite behind it all—starting with its identity and life cycle.
Etiology
What causes malaria, and how does it work its way through the human body?
To understand malaria, we first have to understand its villain—not a virus, not a bacterium, but a parasite. A microscopic, shape-shifting, evolutionary marvel that has been infecting humans for millennia.
This parasite belongs to the Plasmodium genus, and although there are over 100 known species in this group, only five are known to infect humans. Each one has its own quirks, preferences, and levels of danger.
At the top of the list is Plasmodium falciparum, the most dangerous of the bunch. It’s dominant in sub-Saharan Africa and is responsible for the vast majority of malaria deaths worldwide. This species acts swiftly and aggressively, overwhelming the red blood cells and leading to severe disease, including cerebral malaria and multi-organ failure.
Then there’s Plasmodium vivax, which prefers cooler, more temperate climates—regions like Asia and Latin America. It doesn’t usually cause severe illness like falciparum, but it has a unique ability to go dormant in the liver. These “sleeping” parasites, called hypnozoites, can reactivate months later, sparking new rounds of illness even without another mosquito bite.
Plasmodium ovale behaves similarly to vivax, hiding out in the liver and causing delayed relapses. It’s less common and mostly restricted to West Africa, but it deserves attention because of its ability to persist silently.
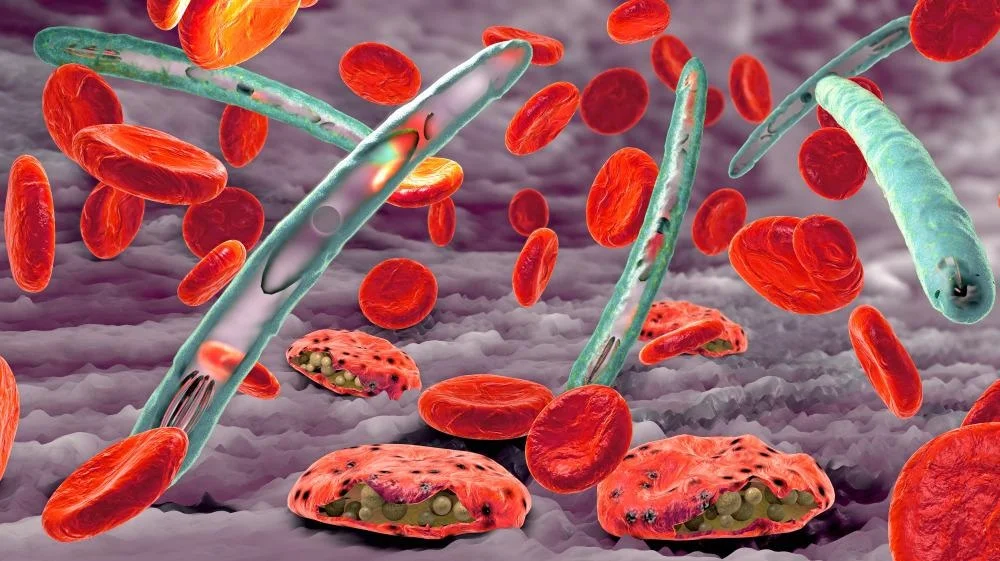
Plasmodium malariae is the quiet one—often overlooked but capable of lingering in the bloodstream for years without causing obvious symptoms. It causes a milder form of malaria but has been known to trigger chronic infections that subtly wear down a person’s health over time.
Lastly, there’s Plasmodium knowlesi, an unusual suspect that originally infected monkeys. In recent decades, it’s made the leap to humans in Southeast Asia. What makes knowlesi alarming is how rapidly it replicates—it can lead to severe disease in a matter of days if not caught early.
But here’s the big question: How do these parasites make us sick?
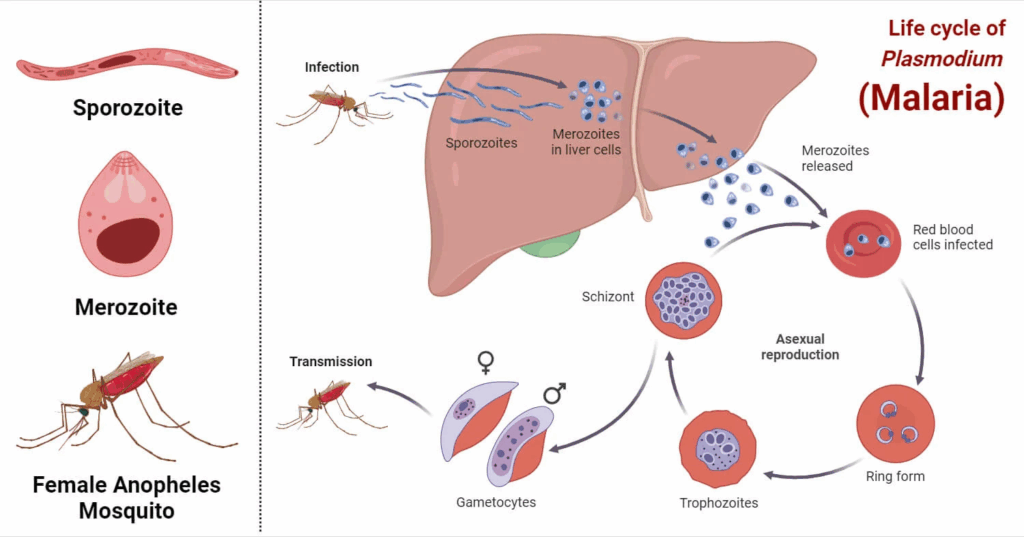
It all starts with a mosquito. Specifically, a female Anopheles mosquito, which acts as both a vector and a host. When she feeds on human blood, she doesn’t just take—she gives. If she’s carrying Plasmodium parasites in her salivary glands, she injects them into the skin along with her anticoagulant-laced saliva. This moment is the beginning of a remarkably intricate biological journey.
Once inside the human body, the parasites—now in their sporozoite form—don’t linger in the blood for long. They quickly make their way to the liver, a critical stronghold in the early stages of infection. Within the liver cells, they begin to multiply silently over the course of about a week or two. This stage is known as the exoerythrocytic (or liver) phase. For most Plasmodium species, this stage ends when the parasites burst out of the liver cells and re-enter the bloodstream. But for vivax and ovale, some parasites take a detour into dormancy, forming hypnozoites that can awaken later—sometimes after weeks, months, or even years.
Once back in the bloodstream, the parasites begin the erythrocytic phase, which is where the clinical symptoms begin. They invade red blood cells, multiply, and then rupture them from the inside—releasing more parasites that go on to infect other red cells. This repeating cycle is responsible for the classic fever spikes and chills that come every 48 to 72 hours, depending on the species. It’s also what causes anemia, jaundice, and, in severe cases, organ damage. The destruction of red blood cells and the immune system’s inflammatory response create a dangerous storm inside the body.
Some of the parasites follow a different path—they develop into gametocytes, the sexual form of the parasite. These gametocytes don’t cause disease in the human host, but they are essential for continuing the transmission cycle. When another mosquito bites the infected person, it picks up these gametocytes, which then mature and reproduce sexually inside the mosquito’s gut. Over the course of 10 to 14 days, the parasite migrates back to the mosquito’s salivary glands, ready to be injected into another unsuspecting host. The cycle has come full circle.
This life cycle is not just fascinating—it’s also strategically important. Each stage presents a potential intervention point. Some drugs aim to kill the parasites in the liver. Others target the blood stage. Newer vaccines are trying to block the parasite from even reaching the liver or from reproducing inside mosquitoes. Insecticide-treated nets and indoor spraying aim to keep mosquitoes at bay altogether, stopping the chain before it can even begin.
But the parasite is a cunning adversary. It changes its surface proteins to confuse the immune system. It hides in the liver or in red cells, cloaked from detection. It adapts to drug pressure, leading to resistance that makes treatment more difficult. Its biological resilience is a key reason why malaria remains such a global threat.
So, when we talk about eradicating malaria, we’re not just talking about killing mosquitoes or handing out pills. We’re talking about dismantling a deeply embedded biological network—one that spans two species, multiple organs, and countless molecular tricks.
Understanding malaria’s etiology—its cause, its parasite, its life cycle—is the first step to defeating it. Now that we’ve seen how the disease takes hold in the body, let’s take a wider view: Where is malaria most common, and who bears the greatest burden?
Epidemiology
Where does malaria thrive, and why are some lives more at risk than others?
To understand how malaria continues to shape global health in 2025, we have to step away from the microscope for a moment and look at the world map. Not a flat political one, but a living, pulsing map—one that breathes with seasons, climate, poverty, migration, and resilience. Malaria doesn’t just exist somewhere. It moves, it clusters, it hides, and sometimes, it surges.
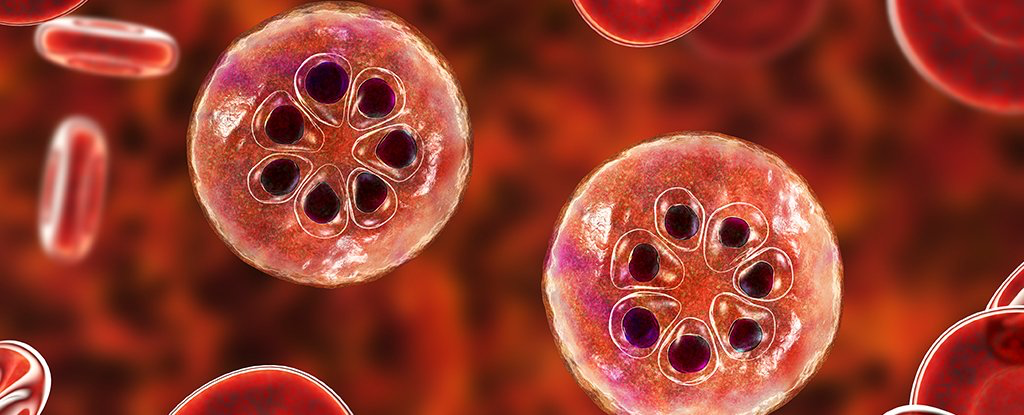
Malaria is not a disease of randomness. It is profoundly geographic, and almost eerily selective. There’s a reason you don’t worry about malaria in Toronto or Tokyo, but it’s a constant presence in rural Uganda or forested parts of Papua New Guinea. The core factor? Mosquitoes—specifically, Anopheles mosquitoes, which are astonishingly climate-sensitive. They need warmth, water, and a bit of human blood. Give them stagnant puddles, humid nights, and a village without window screens or treated nets, and they flourish.
Nowhere is this more evident than in sub-Saharan Africa. In this region, malaria is not just endemic—it’s woven into the rhythm of life. The combination of intense heat, seasonal rains, and a particularly lethal mosquito species, Anopheles gambiae, creates conditions so ideal that malaria doesn’t just survive—it dominates. In these parts, it’s not unusual for a child to have several bouts of malaria a year, their immune system gradually learning, scarred but seasoned. Sadly, many don’t survive long enough to build that immunity. The disease preys on the youngest with a cruelty that’s hard to overstate. Most malaria deaths are children under five, often in rural clinics with little more than hope and paracetamol.
But Africa isn’t the whole story. In parts of South and Southeast Asia, malaria takes on a different shape. It’s less deadly overall, yet more elusive. Plasmodium vivax is the main culprit here—a parasite with a particular taste for mischief. After an initial illness, it can curl up in the liver and go silent, invisible to tests and symptoms, only to reawaken months later when everyone has let their guard down. It’s a different kind of burden—not as brutal as falciparum, but persistent and destabilizing.
And then there’s Latin America, where malaria snakes through jungle rivers and forested borderlands, often striking migrant workers and indigenous communities living far from roads and hospitals. These regions are malaria’s quiet corners—low profile, but no less stubborn. In the Amazon, for example, one might find malaria spreading not through villages, but along logging camps and illegal mining routes, wherever people push into mosquito territory without protection.
What makes this more complicated is that malaria doesn’t just respect geography—it also plays favorites among people. It targets vulnerability. Children are first in its sights, their immune systems too inexperienced to fight it off. Pregnant women are next, their changing physiology making them more susceptible and their unborn children more fragile. Then come the migrants, the refugees, the displaced—the people in motion who are least likely to have a bed net, a nearby clinic, or the means to pay for a diagnostic test. They often sleep under tarps, work at night, and live at the edges of health systems. Malaria, ever opportunistic, finds them.
Interestingly, malaria is a disease that thrives on the margins—not just the physical edges of forests and water, but the social edges too. People living in poverty, in poorly constructed homes, without access to consistent medical care, are the ones who pay its price. Urban centers, with concrete buildings and better infrastructure, usually see lower transmission rates, though not always. Slums on the outskirts of cities can be just as vulnerable as deep rural villages—especially when water collects in containers or clogged drains and the mosquitoes come out at dusk, looking for their next meal.
Even time matters. Malaria follows seasons like a predator follows its prey. In places with a single rainy season, malaria peaks just after the rains, when mosquito breeding explodes. In others with multiple wet seasons, malaria can become a year-round threat, with just enough of a dip between peaks to lull people into complacency. This cyclical pattern means that in some parts of the world, malaria isn’t just a health concern—it’s almost a calendar event.
This is why we can’t talk about malaria without talking about equity. Two children can be born in the same year—one in Oslo, one in Kinshasa—and have utterly different risks of dying from a disease that is entirely preventable and treatable. One may never hear the word “malaria.” The other might grow up seeing the word scribbled on every health clinic wall.
Malaria’s epidemiology is, in a sense, its autobiography. It tells the story of how a parasite found its way into the bloodstreams of the vulnerable, how it shaped and was shaped by colonial histories, economic gaps, environmental shifts, and global priorities. It teaches us that malaria isn’t just a biological problem. It’s a political one. A social one. A climate one. It thrives not just in puddles, but in indifference.
So if we want to know where malaria is—and why it persists—we have to look beyond the microscope. We have to listen to the rain on the metal roof, feel the humidity rise at dusk, and notice which villages don’t have screens on the windows or doctors nearby. That’s where malaria lives. That’s where it waits.
And now that we’ve seen where malaria spreads and who it strikes, the next question is: what happens when it takes hold? What does malaria actually feel like in the body? What are the signs—and why do they differ from one case to another?
Let’s explore that next.
Clinical Features
What does malaria feel like, and why does it affect people so differently?
We’ve talked about the parasite. We’ve followed its journey from mosquito bite to liver cell to red blood cell. But what is it actually like to have malaria?
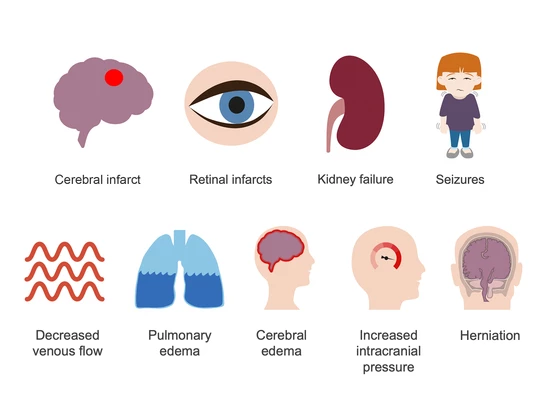
Imagine waking up to a fever that seems to hit like a hammer—hot, relentless, and oddly rhythmic. One moment you’re shivering so violently your teeth clack; the next, you’re drenched in sweat, as if someone poured water over your body. Your head is pounding. Your muscles ache. You feel disoriented, weak, and vaguely nauseous. Time stretches and bends in strange ways when you’re sick like this. The hours blur between bouts of chills, burning heat, and utter exhaustion. This is the classic malaria experience—but it’s far from the only one.
Malaria doesn’t wear just one mask. It’s more like a shapeshifter, a disease of many faces. In some, it comes on like the flu and quietly recedes with treatment. In others, especially when treatment is delayed or inadequate, it transforms into something far more dangerous.
So why the difference? Why does malaria sometimes pass like a bad fever, and other times become deadly?
The answer lies partly in the species of Plasmodium doing the damage. Not all of them behave the same way.
The most notorious—Plasmodium falciparum—is aggressive and fast-moving. It doesn’t just infect red blood cells; it modifies them, making them sticky so they cling to blood vessel walls, including in the brain. This is what can lead to cerebral malaria, a terrifying complication in which seizures, confusion, and coma may develop, particularly in children. Left untreated, it can be fatal within days. And even with treatment, survivors may be left with long-term neurological issues.
P. vivax and P. ovale, in contrast, tend to cause milder disease—though that word can be misleading. Their fevers may be less intense, but they have a cruel trick up their sleeve: latency. After the initial illness, they can vanish into the liver, lying dormant as hypnozoites, then reappear weeks or months later to trigger another bout of fever, fatigue, and malaise. These recurrences can wear down a person’s strength, interfere with school or work, and contribute to chronic anemia—especially in areas where people get infected repeatedly.
P. malariae is quieter still, sometimes lingering in the blood at low levels for years, like a shadow in the background. It may cause only mild symptoms, but over time it can contribute to a kind of slow, smoldering illness. Some patients with chronic P. malariae infection have been found to develop kidney damage—a reminder that even the “mild” species can cause long-term harm.
And then there’s P. knowlesi, the wild card. Though less widespread, it replicates in red blood cells more rapidly than the others—every 24 hours, instead of 48 or 72. This means it can progress very quickly, with fever, chills, and organ involvement escalating in just a few days. Often misdiagnosed as P. malariae under the microscope, it demands careful attention in regions like Malaysia and Borneo.
But species alone doesn’t tell the whole story. The host—the human being—matters just as much. A person’s age, immune status, nutritional state, pregnancy, and history of past malaria exposure all play a role in how the illness manifests.
In areas where malaria is common, children are especially vulnerable. Their immune systems haven’t had the time to build even partial defenses, so they tend to get the worst of it: high fevers, convulsions, vomiting, severe anemia. It’s a leading cause of death in this age group, not just from the parasite itself, but from complications like cerebral malaria, respiratory distress, or overwhelming infection.
Pregnant women face their own set of risks. Malaria during pregnancy can lead to miscarriage, stillbirth, premature delivery, or a baby born with dangerously low birth weight. The placenta becomes a target for the parasite—almost like a Trojan horse—and the results can be tragic.
In contrast, adults who live in high-transmission areas and have had malaria many times often develop semi-immunity. They might still get infected, but they show milder symptoms—or sometimes none at all. This can seem like a blessing, but it creates a hidden danger: asymptomatic carriers who feel fine but can still infect mosquitoes and sustain transmission within a community.
Then there are the travelers—the tourists, backpackers, aid workers, businesspeople who’ve never encountered malaria before. For them, the experience can be shocking. With no prior exposure, the body has no natural defenses. Symptoms can escalate rapidly, and without immediate diagnosis and treatment, falciparum malaria can become a medical emergency in a matter of hours.
So, what do you look for?
It often begins subtly—fatigue, headache, maybe some nausea. Then comes the fever, sometimes spiking in characteristic cycles (every 48 hours with P. falciparum and P. vivax, every 72 with P. malariae). But these cycles are often imperfect, especially early on. Some people think they have the flu. Others might suspect dengue, typhoid, or another tropical illness. That’s what makes diagnosis so challenging—and why a high index of suspicion is crucial in endemic areas.
In severe cases, the symptoms go far beyond fever. There may be confusion, seizures, respiratory distress, shock, kidney failure, or bleeding. At this stage, malaria is no longer just a parasitic infection—it’s a systemic assault.
The variability in symptoms—from recurring fevers to silent relapses—makes malaria a master of disguise. It’s a pattern not unlike what clinicians face with other viral hemorrhagic fevers, where timing and clinical context can completely alter the picture. Lassa Fever, for instance, shares a similar diagnostic challenge: it can present as flu-like in one patient and escalate to organ failure in another, depending on host factors and viral load.
But even the uncomplicated cases can be debilitating. Repeated infections sap strength, disrupt schooling and work, contribute to malnutrition, and reinforce cycles of poverty. A disease doesn’t have to kill to devastate.
In the next section, we’ll ask: how do we actually know when someone has malaria? What tools do we use to confirm it, and how do we tell one species from another? That’s where we turn to diagnostics—sometimes simple, sometimes high-tech, but always a crucial part of the puzzle.
Diagnosis
How do we know it’s malaria? And how can we be sure which kind?
Picture this: a five-year-old child in rural Nigeria is brought into a clinic by her mother. The child has had a fever for two days, she’s not eating, and now she’s started vomiting. She’s listless, a bit confused. It’s the rainy season. The nurse has seen this picture hundreds of times. The first thought? Malaria. But here’s the problem—fever in a child could be anything. Typhoid. Dengue. Viral illness. Even pneumonia. So how do we move from suspicion to confirmation?
Diagnosis is the hinge that everything swings on. If we get it wrong, we waste precious time. We might treat for malaria when it’s actually meningitis—or worse, miss malaria altogether and the parasite keeps destroying red blood cells until the child slips into coma.
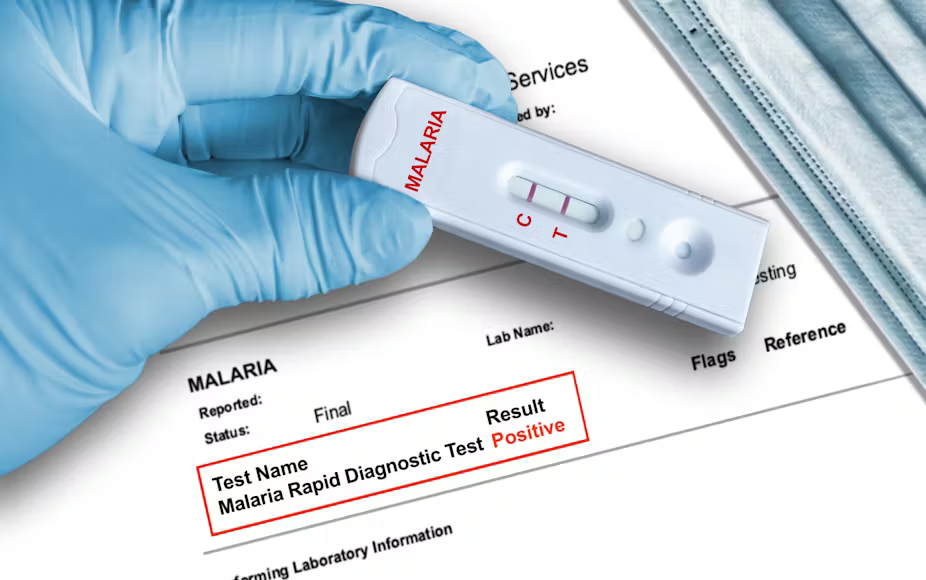
The simplest, oldest, and still most widely used method is microscopy. It’s as low-tech as it is powerful. A drop of blood is placed on a glass slide, stained with a special dye—usually Giemsa—and examined under a microscope by a trained technician. If malaria parasites are present, they become visible, wriggling inside red blood cells like little purple crescents or rings. It’s as much art as science. A good microscopist can not only detect the parasite but estimate how dense the infection is—how many parasites per microliter of blood—and even determine the species. This matters enormously, because the choice of medication can vary depending on whether it’s falciparum, vivax, or something else.
But microscopy, for all its strengths, is not perfect. It requires electricity, a working microscope, fresh reagents, and above all, human expertise. In places where malaria is most common, those things aren’t always guaranteed. That’s where rapid diagnostic tests, or RDTs, have changed the game.
RDTs are small, handheld strips—imagine a pregnancy test, but for malaria. With just a finger-prick of blood and a few drops of buffer solution, the test can detect specific proteins from the malaria parasite—antigens that show up in the blood when the parasite is actively replicating. Within 15–20 minutes, the result appears as a line (or several lines) on the strip. No microscope needed, no lab equipment. It’s fast, easy, and can be done in a remote village under a tree.
But RDTs have their limitations. Many can’t distinguish between different Plasmodium species. Most are optimized for detecting P. falciparum, which is the most dangerous but not the only game in town. Some RDTs also have trouble picking up low-level infections, particularly in patients with partial immunity or chronic, low-density parasitemia. And then there’s the issue of HRP2 gene deletions—a growing concern in certain regions where P. falciparum has evolved to stop expressing the protein that most RDTs rely on to detect infection. In those cases, the test may falsely report “no malaria,” even when parasites are circulating.
So what happens when we need even more precision?
That’s where molecular diagnostics come in. The gold standard is PCR (polymerase chain reaction), which amplifies traces of parasite DNA from a blood sample. PCR is exquisitely sensitive—it can detect even a handful of parasites hiding in the bloodstream. It can also reliably distinguish between species, identify mixed infections, and pick up drug resistance markers. The problem? It’s expensive, slow, and typically limited to research labs or reference hospitals. PCR is not what you reach for in a busy field clinic on a Monday morning.
Still, in specialized settings—like when a traveler returns to the U.S. or Europe with unexplained fever, or when researchers want to map the true burden of asymptomatic malaria in a population—molecular tools are invaluable. They give us a much deeper, more nuanced picture of transmission than a quick blood smear ever could.
There’s also growing interest in next-generation diagnostics. These include loop-mediated isothermal amplification (LAMP), which offers PCR-like sensitivity with simpler equipment. There’s even talk of CRISPR-based diagnostics on the horizon—where small gene-editing systems can be used not to cut DNA, but to glow when they find malaria genes. It’s science-fiction made real. But we’re not quite there yet at scale.
Then there’s the human factor. Clinical diagnosis, meaning diagnosis based only on symptoms and context, is still heavily relied upon—especially in overstretched clinics or during outbreaks when supplies are low. But it’s a double-edged sword. In high transmission zones, almost every child with a fever is assumed to have malaria. Sometimes that assumption is right. But other times, it blinds health workers to other deadly causes of fever—like sepsis or meningitis. Misdiagnosis can be fatal in either direction.
So what’s the best path? Ideally, it’s a layered approach. If microscopy is available and reliable, use it. If it’s not, RDTs are often the next best thing. Molecular methods fill in the gaps for complex cases or for epidemiological clarity. And all of these tools work better when supported by training, good logistics, and a health system that can respond not just with a diagnosis—but with treatment.
In a perfect world, every person with a fever in a malaria-endemic region would get a high-quality diagnostic test within minutes of walking into a clinic. No guessing. No delay. Just answers. But until we reach that ideal, the battle to diagnose malaria remains as much about access as it is about technology.
And once the diagnosis is made, we arrive at the next, most urgent question: how do we treat it—and how do we deal with the growing problem of drug resistance?
We’ll take that on next.
Treatment
So you’ve got malaria—now what? Can it be cured? And what happens if the medicine doesn’t work anymore?
The moment a clinician confirms malaria—whether through a blood smear under a microscope, a rapid diagnostic test, or molecular detection—it feels like crossing a line. Now we know what we’re dealing with. But the next question is often more urgent, and far more personal: Can it be treated? Can I be cured?
The good news is yes—malaria is treatable. In fact, for all its complexity, malaria is one of the few tropical diseases for which we have a clear cure, at least for most cases and most people. But that doesn’t mean the path is always simple.
Let’s start with Plasmodium falciparum, the most dangerous and most widespread form, especially in sub-Saharan Africa. The frontline weapon here is artemisinin-based combination therapy, or ACTs. These combinations are like a one-two punch: artemisinin works fast, wiping out most of the parasites within the first 48 hours, while the second drug lingers longer in the bloodstream to mop up the rest. This dual approach is designed to both cure the patient and prevent the parasites from developing resistance.
ACTs changed the game when they were introduced. Before artemisinin, the world relied heavily on chloroquine, but by the 1990s, falciparum had developed widespread resistance to it—especially in Africa and Asia. In many cases, patients would take the drug, start to feel better, only to relapse or even die because the parasite had become unresponsive. ACTs turned that tide, and for years, they’ve been incredibly effective.
But the story doesn’t end there. In parts of Southeast Asia, especially along the Thailand-Cambodia border—a region historically known as the “cradle of resistance”—there have already been reports of ACT resistance. Parasites have begun to mutate in ways that make them slower to clear from the bloodstream, even when given full doses. This doesn’t mean ACTs are useless, but it’s a red flag, a warning that the parasite is evolving again.
If ACTs fail, treatment becomes more complicated. In severe cases—especially those involving cerebral malaria, organ dysfunction, or extremely high parasite loads—the oral route is no longer enough. Patients need intravenous therapy, usually with IV artesunate, a powerful derivative of artemisinin given over several days. This treatment is often administered in hospitals or specialized clinics and requires careful monitoring, especially for side effects like hemolysis (the breakdown of red blood cells), which can occur days after the treatment ends.
But what about the other species?
For Plasmodium vivax and Plasmodium ovale, ACTs can treat the immediate infection, but they can’t touch the hypnozoites—those dormant liver stages that can reactivate weeks or months later. To truly cure vivax or ovale, you need a second medication: primaquine. It’s the only widely available drug that targets the liver stage and prevents relapse. But primaquine comes with a catch—it can cause a dangerous breakdown of red blood cells in people with G6PD deficiency, a common genetic condition in many malaria-endemic populations. That means patients need to be screened before taking it—something that isn’t always feasible in rural or under-resourced settings. Newer alternatives like tafenoquine, a single-dose liver-stage drug, offer hope for easier treatment, but they come with the same G6PD caveat.
P. malariae and P. knowlesi usually respond to ACTs or even older drugs like chloroquine—though care must be taken with knowlesi, which can progress rapidly. Because it replicates faster than the others, patients can deteriorate quickly, and treatment must begin as soon as possible after diagnosis.
Now, let’s ask the uncomfortable question: What happens when treatment fails?
There are several reasons why a patient might not recover. Sometimes, it’s delayed treatment—the infection has progressed too far, the parasite load is too high, or vital organs have already been compromised. Other times, it’s substandard or counterfeit medications—a massive and underreported problem in some parts of the world, where supply chains are weak and regulation is lacking. Pills may be expired, improperly stored, or even completely fake. And then there’s drug resistance, the looming threat that keeps global malaria programs awake at night.
Resistance doesn’t usually explode overnight. It creeps in. It begins with a few treatment failures, then more, then suddenly a region is facing parasites that don’t respond to the standard drugs. Resistance to chloroquine swept through Asia and Africa like wildfire in the 20th century. If full resistance to ACTs takes hold, particularly in Africa, the consequences could be catastrophic—millions of lives at risk, decades of progress undone.
That’s why treatment isn’t just a clinical question—it’s a strategic, global one. Monitoring drug effectiveness, rotating therapies, investing in new compounds, and preventing transmission all become part of a delicate balancing act. One poorly managed outbreak in a remote village can become the seed of global resistance if given enough time and mobility.
Meanwhile, patients don’t think in terms of drug policy or molecular pathways. They think in terms of suffering, and relief. A child who gets ACTs, feels better in 24 hours, and goes back to school the next week—that’s the real goal. Treatment isn’t just about biochemistry. It’s about restoring the rhythm of life.
So yes, malaria is treatable. Often curable. But never simple. Every successful treatment is a little miracle of access, logistics, molecular biology, and trust. And those miracles need to keep happening, every day, in thousands of clinics around the world.
But what if we didn’t have to treat so many people in the first place? What if we could stop malaria before it starts?
That’s the promise of prevention—the next stage in this story.
Prevention
What if we could stop malaria before it even enters the body? Can we really keep the mosquito—and the parasite—at bay?
The old saying goes: “An ounce of prevention is worth a pound of cure.” With malaria, that ounce could mean a bed net. Or a pill. Or a wall sprayed with insecticide. Or—perhaps someday—a vaccine. But prevention, like everything else in the malaria story, is not one-size-fits-all. It’s layered, evolving, and in many cases, deeply local.
Let’s start with the mosquito, because that’s where malaria begins. Without her—the Anopheles mosquito—Plasmodiumhas no vehicle, no wings, no bite. So much of malaria prevention has focused on controlling mosquito populations and interrupting the contact between mosquito and human.
The most iconic tool in this fight is the insecticide-treated bed net. At first glance, it seems deceptively simple—just mesh draped over a sleeping space. But it’s also a technological marvel: treated with long-lasting insecticides, these nets do double duty. They act as a physical barrier, preventing mosquitoes from getting close, and a chemical one, killing or repelling them on contact. The result? Massive reductions in transmission, especially when coverage is high.
Nets have been so effective, in fact, that their widespread distribution is often credited with millions of averted cases and deaths over the past two decades. In many parts of Africa, they’re as essential as food and water—hung over beds, cribs, even hammocks. But they aren’t perfect. Nets can tear. Insecticides can fade. And mosquitoes are adapting, biting earlier in the evening or outdoors, where nets offer no protection. Some mosquito populations have even developed resistance to the insecticides themselves.
Which brings us to the second line of defense: indoor residual spraying, or IRS. Here, walls inside homes are sprayed with powerful insecticides that linger for weeks or months. When mosquitoes land on these surfaces, they absorb a fatal dose. It’s a method that’s especially useful in areas with seasonal transmission—spray just before the rains come, and you can dramatically reduce malaria risk for the entire community.
IRS, however, is resource-intensive. It requires teams of trained sprayers, access to every household, and community cooperation. People must allow strangers into their homes with canisters of chemicals—and that’s not always an easy sell, especially in places where trust in health authorities is fragile. Like nets, IRS also faces the challenge of insecticide resistance, which means that programs must continually adapt and rotate their chemicals to stay ahead of the mosquitoes.
But what if you’re not at home? What if you’re a traveler, a forest worker, or someone displaced by conflict?
That’s where chemoprophylaxis comes in—the use of preventive antimalarial drugs. For travelers from non-endemic countries visiting malarial zones, prophylaxis is standard. Drugs like atovaquone-proguanil, doxycycline, or mefloquineare taken before, during, and after the trip to suppress any parasites that might sneak into the bloodstream. When taken properly, they’re highly effective—but they come with side effects, and adherence can be tricky, especially when the schedule continues for weeks after returning home.
In endemic regions, chemoprophylaxis plays a different role. It’s not about tourists—it’s about targeted protection for the most vulnerable. Pregnant women, for example, may receive intermittent preventive treatment in pregnancy (IPTp)—regular doses of antimalarial drugs during routine antenatal visits. Similarly, in high-risk areas, children may receive seasonal malaria chemoprevention (SMC): monthly doses of preventive medicine during the rainy season, when transmission peaks.
These approaches don’t eliminate the parasite, but they buy time—time for immunity to build, for vaccines to take hold, for health systems to catch up.
Which brings us to the long-awaited promise: vaccines.
For decades, a malaria vaccine was something of a holy grail—a near-impossible dream, given how clever and complicated the parasite is. Plasmodium has dozens of stages in its life cycle, each with different surface proteins. It mutates, hides, and misleads the immune system. Designing a vaccine against it is like trying to hit a moving target, blindfolded.
But the dream is no longer fiction.
In 2021, the World Health Organization endorsed the RTS,S/AS01 vaccine—the first malaria vaccine ever recommended for widespread use. It’s not perfect. It doesn’t provide sterilizing immunity. Efficacy hovers around 30–50%, depending on the age group and location. It requires four doses. But it works. In pilot programs across Ghana, Kenya, and Malawi, RTS,S was shown to significantly reduce severe malaria and hospitalizations among children—especially when used alongside existing tools like nets and ACTs.
And now, there’s more. In 2023, the R21/Matrix-M vaccine received regulatory approval. Early data suggests it may offer higher efficacy and be easier to manufacture at scale. It’s too soon to declare victory, but this moment marks a turning point. For the first time, multiple vaccines are on the table—and that changes everything.
Still, prevention isn’t just about tools. It’s about people—how communities engage with malaria, how they perceive risk, and how they integrate prevention into daily life. A bed net that stays folded in its bag helps no one. A child who skips their SMC dose remains vulnerable. A family who distrusts the spray team may be left exposed.
Malaria prevention is a dance between science and society. It takes more than medicine—it takes mobilization, trust, and persistence. We can kill the mosquitoes, block the parasites, and immunize the children—but we have to do it again, and again, and again, because malaria is nothing if not relentless.
And yet, the tide may be turning. Insecticides are being reformulated. New vaccine candidates are emerging. Communities are being empowered. Global investment, while fragile, still fuels the engine.
So yes, malaria can be prevented. Not perfectly, not permanently—not yet. But every net hung, every dose given, every house sprayed, and every vaccine administered is another stitch in a global safety net that’s slowly, steadily being woven.
Next, we’ll look at how far we’ve come in just the last year or two. What’s new? What’s hopeful? And what still keeps malaria specialists up at night?
Recent Developments (2025–2026)
Are we finally turning a corner? What’s new in the fight against malaria—and what could change everything?
Every year, malaria researchers, public health workers, and policy leaders ask themselves the same question: Are we making progress? And every year, the answer is complicated. In some ways, malaria is more fragile than ever—on the ropes, pressured from multiple sides. But in others, it’s adapting, reasserting itself, and finding new ways to survive.
So what happened in the last year or two? A lot. Quietly, sometimes unevenly, but undeniably, the malaria landscape has begun to shift again.
Let’s start with the one word that has long loomed like a beacon in this field: vaccines.
In late 2023, the new R21/Matrix-M vaccine entered the scene—not with the fanfare of a miracle drug, but with the quiet, thrilling authority of data. Early trials had suggested a higher efficacy than the first-generation RTS,S vaccine, and those hopes have begun to bear out in real-world settings. Now, in 2025, R21 is being rolled out in a phased manner across several African countries, particularly in high-burden zones where existing tools alone haven’t been enough to curb mortality. There’s cautious optimism among experts—not because this vaccine ends the story, but because it might shift the odds. When used in tandem with nets, sprays, ACTs, and SMC programs, even a modestly effective vaccine could tip the scales in favor of survival.
And here’s what’s even more interesting: for the first time, manufacturing capacity is being scaled up within Africa itself. Vaccine doses are being produced not just in Europe or India, but in Africa, for Africa. That kind of sovereignty doesn’t just save money—it saves time, and builds resilience.
But vaccines aren’t the only frontier. On the vector control side, we’re entering what might be described as a quiet arms race—with scientists on one side, and mosquitoes on the other. One of the most significant recent advances is the deployment of next-generation insecticides—especially those in the chlorfenapyr and clothianidin classes. These are designed to outmaneuver the resistance that mosquitoes have developed to older compounds like pyrethroids. New nets are being trialed and distributed that contain dual-active ingredients, aimed at killing even the resistant strains. Field results are promising, showing better mosquito mortality and improved protection in areas where standard nets had started to lose their punch.
Meanwhile, in the shadows of cutting-edge labs, another revolution is quietly brewing: gene-drive technology. This approach doesn’t aim to kill mosquitoes with chemicals but to genetically alter their ability to carry malaria parasites, or even to reproduce altogether. The idea is breathtaking in its ambition: release a few modified mosquitoes into the wild, and over several generations, they pass on the altered genes, collapsing the population or rendering it harmless. It sounds like science fiction. But field trials are already underway in tightly controlled environments. Regulatory and ethical concerns abound—as they should—but the potential is staggering. Imagine if the very mosquito that’s been malaria’s enabler for centuries could be flipped into a dead end for the parasite.
Technology is moving forward, too, on the diagnostic front. We’ve seen advances in CRISPR-based testing, which promises the sensitivity of PCR with the speed of a rapid test. Researchers are developing handheld devices that could identify malaria DNA in a drop of blood in under 30 minutes—with no need for a cold chain, electricity, or even skilled technicians. If these tools become affordable and scalable, they could redefine diagnosis in rural clinics where speed and accuracy mean life or death.
But not all the news is upbeat.
2025 has also been a year of vigilance—particularly in the Greater Mekong Subregion, where artemisinin resistance continues to spread like a slow-burning fire. Some ACTs that once cleared infections in 48 hours are now taking longer, and in some cases, failing outright. Containment efforts are being pushed hard, but the worry is always the same: if resistance jumps continents—especially to Africa—it could undo years of hard-fought gains. That scenario isn’t inevitable, but it’s a real and urgent threat.
Climate, too, is now in the malaria conversation in a way it never was before. Rising temperatures and changing rainfall patterns are shifting the geography of malaria in real time. Places that were once too cool or dry are becoming more mosquito-friendly. Highland areas of East Africa, once relatively malaria-free, have started to see seasonal transmission. The disease is creeping into new zones, catching communities off guard. These aren’t anomalies—they’re previews of what may become the new normal.
And finally, there’s the human element—the policies, the money, the political will. Global malaria funding plateaued in the early 2020s, and while there’s been a slight rebound post-COVID, the gap between what’s needed and what’s available remains significant. Some countries are shouldering more of the cost, which is encouraging, but many still rely heavily on international donors. The fear is that as malaria becomes less visible—less of a headline—the urgency may fade. But malaria doesn’t fade. It waits. And when programs falter, it returns.
So what’s the takeaway from 2025–2026?
We’re seeing a complex picture. On one hand, tangible progress: new vaccines, better insecticides, smarter diagnostics, even the beginnings of genetic tools that could change the game. On the other, emerging threats: resistance, funding gaps, climate unpredictability, and persistent inequality. It’s a race—between innovation and adaptation, investment and apathy.
And yet, the progress matters. For the child who didn’t get sick because she got her SMC dose on time. For the clinic that had nets to distribute and medicine to treat. For the researcher who sees the parasite under a microscope and knows the next generation might not.
In the next and final section, we’ll pull the lens back one last time and ask the biggest questions of all: Where are we going? Can malaria ever truly be eliminated? And what will it take to get there?
Frequently Asked Questions (FAQ)
1. What exactly causes malaria?
Malaria is caused by Plasmodium parasites, single-celled organisms transmitted to humans through the bite of an infected female Anopheles mosquito. Five species affect humans, with Plasmodium falciparum being the deadliest.
2. How does the malaria parasite move through the human body?
After a mosquito bite, the parasite travels from the bloodstream to the liver, where it multiplies silently. Then it bursts into the bloodstream, invading red blood cells, reproducing, and destroying them in cycles. This is what causes fever and complications.
3. Where in the world is malaria most common?
Malaria is most prevalent in sub-Saharan Africa but is also found in parts of South Asia, Southeast Asia, Latin America, and Oceania. It thrives in warm, humid climates with standing water—ideal conditions for mosquito breeding.
4. Who is most at risk of getting severe malaria?
Children under five, pregnant women, immunologically naïve travelers, and displaced populations are most at risk. Poverty and limited access to healthcare also greatly increase vulnerability.
5. What does malaria actually feel like?
Malaria typically causes cyclical high fevers, chills, sweating, headache, nausea, and fatigue. Severe cases can lead to confusion, seizures, anemia, respiratory distress, and organ failure—especially with P. falciparum.
6. How is malaria diagnosed?
Diagnosis can be done by examining blood under a microscope, using rapid diagnostic tests (RDTs), or more advanced methods like PCR. Each method has strengths and weaknesses depending on the setting and the resources available.
7. Can malaria be cured?
Yes, malaria is treatable and curable. Artemisinin-based combination therapies (ACTs) are the frontline treatment for P. falciparum. Other species may require additional drugs like primaquine to target liver stages and prevent relapse.
8. What happens if the parasite becomes resistant to treatment?
Drug resistance, especially to ACTs, is a growing concern in parts of Southeast Asia. If resistance spreads widely, especially to Africa, it could reverse decades of progress. Monitoring and new drug development are essential.
9. How can malaria be prevented?
Prevention strategies include insecticide-treated bed nets, indoor residual spraying, seasonal chemoprevention for children, prophylactic drugs for travelers and pregnant women, and now, vaccines like RTS,S and R21.
10. What’s new in malaria prevention and control?
Recent advances include the rollout of new vaccines, deployment of next-gen insecticides, development of gene-drive mosquitoes, and more precise diagnostic tools. Simultaneously, challenges like resistance, climate change, and funding shortfalls remain.
Conclusion
Where are we going—and can malaria ever truly be defeated?
It’s tempting to think of malaria as a problem of the past. After all, we’ve come a long way. In many countries, malaria used to be part of daily life—an unquestioned rhythm of sickness and survival. Today, in dozens of those places, it’s gone. Eliminated. That’s progress.
But for hundreds of millions, malaria is still a daily possibility, a hovering threat, a reality that shapes how people sleep, where they live, when they work, and whether their children survive. Despite its ancient roots, malaria is not fixed in the past. It’s happening now.
And yet—we’re not where we were twenty years ago. We’re not even where we were five. The tools have grown sharper. The science is accelerating. Vaccines once thought impossible are rolling out in clinics. Mosquitoes are being genetically reprogrammed. Satellite data helps predict outbreaks. Community health workers are delivering life-saving care to the most remote corners of the world. That matters. That’s not a small shift—it’s a tectonic one.
But malaria won’t go quietly. The parasite is clever. The mosquito is resilient. Resistance is always a step away. And funding, political will, and public attention are, as always, fragile. Success here is not just about killing a parasite—it’s about holding a line, year after year, until that parasite has nowhere left to go.
As malaria pushes into new territory due to climate shifts and population movement, it joins a growing list of diseases that defy old geographic assumptions. Avian Influenza H5N1 is a case in point—once thought to be tightly regional, it’s now a global concern, showing how fast a localized threat can ripple outward if the conditions are right.
Will malaria be eliminated in our lifetime?
It’s possible. Not inevitable—but possible. The tools are here. The knowledge is here. The challenge is sustaining the effort, expanding the reach, and refusing to settle for “good enough.”
Malaria is a disease of biology, yes. But more than that, it is a disease of inequity. Where it thrives, so often, is where systems fail. And so, the fight against malaria is also a fight for justice—for a world where birth geography no longer dictates the odds of survival.
In the end, this is not just a story about a parasite and a mosquito. It’s about humans. And whether we choose, collectively, to finish the work we started.