H1N1 Influenza with Drug Resistance: Challenges and Strategies
Introduction
What comes to mind when you hear “H1N1”? A pandemic? A flu shot you skipped last year? Or maybe just another virus in the alphabet soup of global health threats? However familiar or unfamiliar it may sound, H1N1 influenza is far more than a seasonal nuisance. It’s a pathogen with a dramatic history, a complex biology, and—perhaps most pressing—a knack for outsmarting some of our best pharmaceutical defenses.
That dynamic isn’t unique to H1N1; in fact, it shares conceptual ground with other high-consequence pathogens like the Marburg virus, which similarly challenge our diagnostic speed and therapeutic limits.
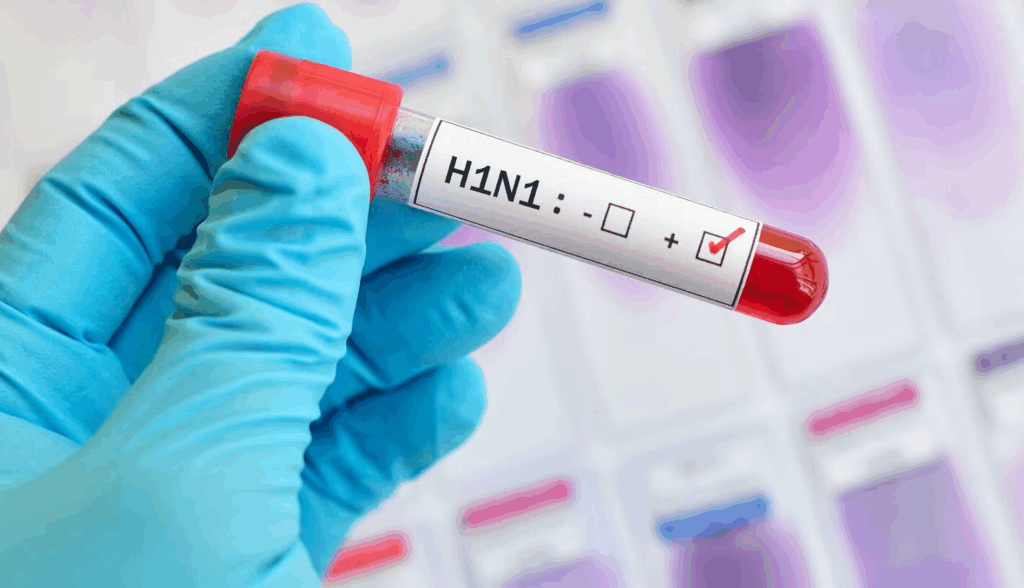
But let’s step back for a moment. Why does H1N1 deserve such sustained attention in the 2020s, especially when so many other viruses—SARS-CoV-2, for instance—have dominated headlines? The answer lies not only in its past but in the evolving challenge of drug resistance. If you’re reading this, you’re probably already aware that drug-resistant infections aren’t just hypothetical. They’re real, they’re rising, and they’re redefining how we think about viral treatment in both clinical and public health settings.
A Quick Overview: What Is H1N1, Exactly?
H1N1 is a subtype of the influenza A virus, one of the main culprits behind seasonal flu epidemics and occasional pandemics. The designation “H1N1” refers to the specific combination of two surface proteins: hemagglutinin type 1 (H1) and neuraminidase type 1 (N1). These proteins are more than just viral décor—they’re critical to the virus’s ability to infect cells and spread within the human body.
Influenza A viruses, including H1N1, are RNA viruses, which means they replicate rapidly and mutate frequently. This high mutation rate makes them a moving target for the immune system and for drug developers. It’s why last year’s flu vaccine might not fully protect you this year. And it’s also why antiviral resistance can emerge so swiftly.
But what sets H1N1 apart from other flu strains? For starters, it’s been the source of at least two major global health events: the 1918 “Spanish flu” pandemic—yes, that was an H1N1 virus—and the more recent 2009 “swine flu” pandemic. Both events demonstrated how swiftly and unpredictably influenza viruses can spread, adapt, and cause widespread disruption.
The Historical Backdrop: From Spanish Flu to Swine Flu
To grasp the public health weight of H1N1, it helps to revisit its dramatic debut. The 1918 pandemic was catastrophic, killing an estimated 50 million people worldwide. Interestingly, it hit young, otherwise healthy adults the hardest—a grim reversal of the typical flu pattern, which tends to spare the young and claim the old. The cause? A hyper-aggressive immune response called a cytokine storm, which we’ll explore in more depth later.
Fast-forward to 2009. A genetically novel H1N1 virus emerged, bearing genetic segments from human, avian, and swine influenza strains—a “quadruple reassortant,” if you want the virological term. It quickly triggered a pandemic, though this time, fortunately, the mortality rate was far lower than in 1918. Still, the 2009 pandemic was a wake-up call. It revealed how interconnected and vulnerable our world had become, and it exposed serious gaps in global pandemic preparedness.
Even after the WHO declared the official end of the 2009 pandemic in 2010, the H1N1 virus didn’t vanish. Instead, it settled in. It became part of the seasonal flu landscape, quietly evolving and circulating alongside other influenza strains.
Why Drug Resistance Matters — Now More Than Ever
Now here’s where things start to get really concerning. In the post-pandemic years, H1N1 viruses have increasingly shown resistance to oseltamivir (Tamiflu)—one of the most commonly prescribed antivirals for influenza. Initially, oseltamivir resistance was rare and isolated. But like bacteria with antibiotics, viruses under drug pressure can evolve. A single mutation in the N1 gene (H275Y, for those interested) can significantly reduce the drug’s effectiveness.
Why does this matter? Because antivirals aren’t just “nice to have.” They’re critical tools in controlling outbreaks, especially in vulnerable populations or when vaccines are poorly matched to circulating strains. A virus that shrugs off our best antivirals forces us into a much more precarious defensive position.
And if H1N1 continues to develop and spread resistant variants, we may find ourselves confronting not just another flu season—but a full-blown therapeutic crisis.
A Living Virus in a Changing World
The story of H1N1 is far from over. It’s a virus that continues to evolve, adapt, and challenge our assumptions about influenza control. It lives at the intersection of virology, immunology, global health, and policy. And when drug resistance enters the mix, it forces an uncomfortable question: Are we truly prepared for the next wave?
In the chapters that follow, we’ll explore the virus from the inside out—its structure, its spread, how it affects the body, how we diagnose it, treat it, and try to stay a step ahead. We’ll examine recent developments and strategies for prevention and control. And yes, we’ll confront the increasingly urgent problem of antiviral resistance with both a scientific lens and a practical one.
But let’s step back for a moment. Why does H1N1 deserve such sustained attention in the 2020s—especially when so many other viruses, like Mpox, have re-emerged from the periphery of public health concern?
So whether you’re a student, a clinician, a public health worker, or just an intellectually curious reader—you’re in the right place. Let’s dive into what makes H1N1 such a formidable viral adversary, and why understanding it deeply is essential in the age of emerging infectious diseases.
Virology and Pathogenesis
If you were to shrink down to the microscopic scale and come face-to-face with the H1N1 influenza virus, what would you actually see? Would it look dangerous? Malevolent? Probably not. It would seem rather unassuming—spherical, studded with little protein spikes, and barely 100 nanometers in diameter. But don’t be fooled by appearances. This tiny viral particle packs an extraordinary punch, equipped with a toolkit of genetic tricks and molecular weapons that enable it to hijack your cells, evade your immune system, and replicate with astonishing speed.
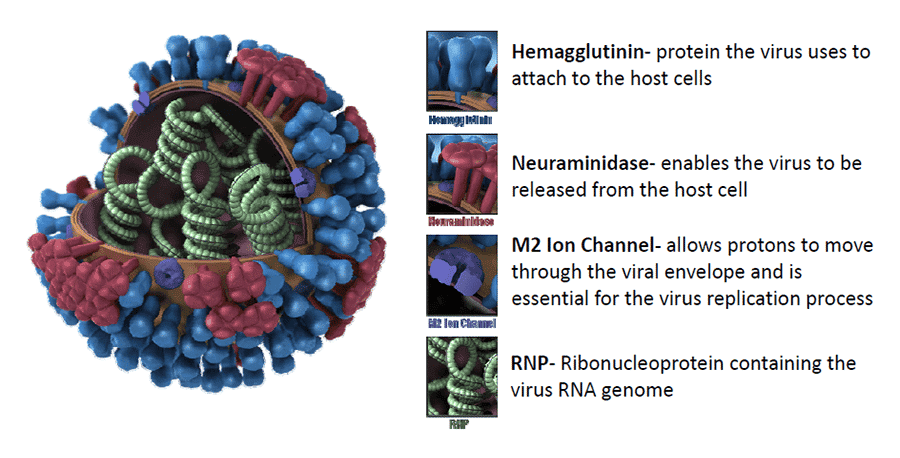
What’s Inside the Virus? Understanding Its Architecture
Let’s start with the basics. H1N1, like all influenza A viruses, has a lipid envelope—a sort of oily outer coat derived from the host cell’s membrane. Embedded in that envelope are the two most important surface proteins we briefly mentioned earlier: hemagglutinin (HA) and neuraminidase (NA). These are the H and N in H1N1.
But what do they actually do?
- Hemagglutinin (H1) is the key that unlocks the door. It binds to sialic acid receptors on the surface of human respiratory cells, allowing the virus to attach and initiate entry.
- Neuraminidase (N1) is the escape artist. After the virus replicates and new viral particles are assembled inside the host cell, NA helps them break free by cleaving sialic acid residues. Without it, the newly formed viruses would get stuck to the host cell or to each other.
These proteins are also the primary antigenic targets—meaning they’re what your immune system “sees” and remembers after an infection or vaccination. Unfortunately, they’re also prone to mutation, which is why flu viruses change so frequently, and why our vaccines often play catch-up.
Beneath the surface, the H1N1 virus carries eight single-stranded RNA segments, each encoding one or more viral proteins. This segmented genome is what allows influenza to perform its infamous genetic shuffle, known as reassortment—a mechanism that can create entirely new viral strains when different influenza viruses co-infect the same host cell.
How the Virus Invades and Replicates: A Molecular Heist
So what happens once H1N1 breaches your respiratory system? The invasion begins in the upper airway—the nose, throat, and bronchi. The HA protein latches onto epithelial cells, triggering endocytosis—the process by which a cell engulfs an external particle.
Inside the host cell, the virus sheds its coat and releases its RNA segments. These segments are transported into the nucleus (unusual for an RNA virus, by the way) where they’re transcribed and replicated using the host’s own cellular machinery. It’s a viral takeover, clean and efficient. Proteins are synthesized, new RNA genomes are assembled, and hundreds of progeny viruses are churned out within hours.
Eventually, the cell is overwhelmed. It may die outright or explode (a process called lysis), releasing viral offspring to infect neighboring cells. Meanwhile, the immune system is scrambling to catch up.
Immune Response: Balancing Defense and Destruction
Now let’s talk about the immune system—your body’s internal security force. Once it detects the infection, it launches a multi-layered response. Innate immunity kicks in first, with interferons, cytokines, and natural killer cells trying to curb the spread. If that initial defense falters, the adaptive immune system mobilizes—B cells begin producing antibodies against HA and NA, and T cells target infected cells for destruction.
In most people, this coordinated response controls the infection within a few days. But here’s where things can go wrong.
Sometimes, the immune system overreacts—especially in young, healthy adults with robust immune systems. It releases a storm of inflammatory signals (cytokines), leading to acute respiratory distress, lung damage, and, in severe cases, death. This phenomenon, the infamous cytokine storm, was partly responsible for the high mortality in the 1918 pandemic.
And in other individuals—particularly the elderly, immunocompromised, or those with chronic illnesses—the immune response might be too slow or too weak, allowing the virus to replicate unchecked and increasing the risk of secondary bacterial infections like pneumonia.
Why Mutations Matter: Antigenic Drift and Shift
Here’s where influenza’s trickery really comes into focus. The virus constantly mutates through a process known as antigenic drift—small, gradual changes in the HA and NA proteins that accumulate over time. These minor tweaks can help the virus evade pre-existing immunity and reduce the effectiveness of vaccines.
But sometimes, the virus goes even further. When two different influenza viruses infect the same host, their RNA segments can reassort, producing a new hybrid virus with a novel HA and/or NA combination. This is called antigenic shift, and it’s how pandemic strains like the 2009 H1N1 emerge. The human immune system has little or no prior exposure to such combinations, allowing the virus to spread rapidly on a global scale.
Drug resistance can evolve through both processes. For instance, a single point mutation in the NA gene—like H275Y—can reduce the binding affinity of neuraminidase inhibitors like oseltamivir, making treatment less effective or even useless. These mechanisms echo what we’ve seen in other influenza strains, including Avian Influenza H5N1, where genetic drift and zoonotic spillovers add further layers of unpredictability to an already slippery adversary.
Viral Fitness: Can a Resistant Virus Still Thrive?
You might wonder: if resistance mutations impair a drug target, don’t they also hurt the virus? That’s a great question—and for a time, that was the optimistic view. Some resistance mutations do reduce viral fitness, making the virus less capable of replication or transmission.
However, viruses are resourceful. They often acquire compensatory mutations elsewhere in the genome that restore or even enhance fitness. That’s what we’ve observed in some H1N1 variants: they carry resistance mutations without sacrificing transmissibility, meaning they can spread through the population even in the absence of drug pressure.
In other words, resistance doesn’t necessarily come at a cost. Sometimes, the virus gets to have its cake and eat it too.
Epidemiology
So where exactly does H1N1 hang out when it’s not making headlines? Does it lurk in specific regions, or is it a global nomad? And why do some populations seem to get hit harder than others?
To understand the threat H1N1 poses—and how drug resistance factors in—we need to know how this virus behaves in the real world. This means looking not just at virology under a microscope, but at patterns in populations: geography, seasonality, demographics, and social factors all play critical roles in how the virus spreads and how outbreaks evolve.
A Truly Global Virus: Distribution and Persistence
Unlike some infectious diseases that are confined to specific regions—think malaria or yellow fever—H1N1 is truly cosmopolitan. It circulates globally, often without regard for borders or climate zones. Since the 2009 pandemic, H1N1 has become one of the dominant strains in seasonal influenza epidemics across every continent except Antarctica.
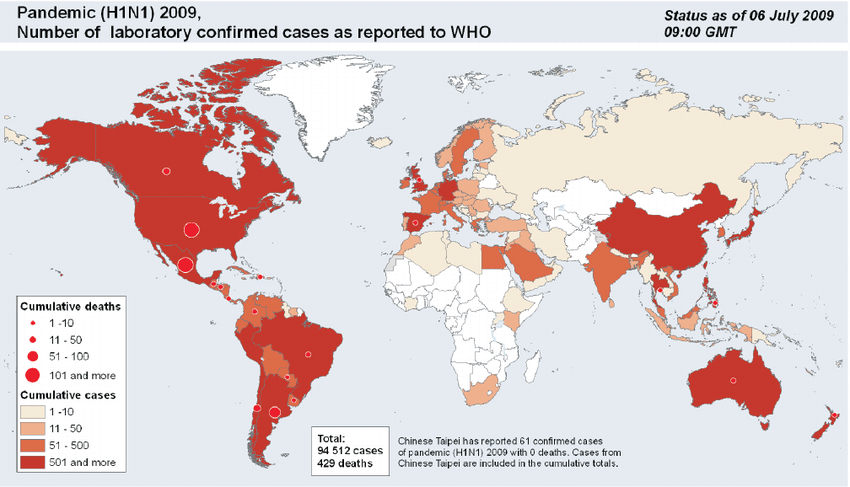
In the post-pandemic years, H1N1 hasn’t gone away; it has simply integrated itself into the seasonal flu pattern, co-circulating with H3N2 and influenza B. In some years, H1N1 predominates. In others, it plays second fiddle. But make no mistake—it’s never far from the stage.
Importantly, surveillance data from the WHO and national health agencies show that H1N1 activity tends to spike in winter months in temperate regions (December to March in the Northern Hemisphere, June to September in the Southern). In tropical regions, however, influenza—including H1N1—may circulate year-round, often in bursts aligned with rainy seasons or social behaviors like school terms.
Who Gets Sick: Age, Risk, and Vulnerability
One of the more curious features of the 2009 H1N1 pandemic was its unusual impact on younger adults. Unlike typical seasonal flu, which hits the elderly and very young hardest, H1N1 in 2009 caused significant illness and even death among people in their 20s, 30s, and 40s.
Why? One theory is immunological naivety: older adults may have had partial immunity due to prior exposure to H1N1-like viruses earlier in the 20th century, while younger individuals did not. It’s a reminder that age doesn’t always map neatly onto vulnerability—immunological history matters, too.
Today, the most affected groups include:
- Children under 5 years old, whose immune systems are still developing.
- Pregnant individuals, who face altered immune function and higher risk of complications.
- Elderly adults, especially those over 65, due to weakened immune responses.
- People with chronic conditions, such as asthma, diabetes, heart disease, or immunosuppressive disorders.
- Healthcare workers, who face repeated exposure.
When H1N1 infections occur in these groups, outcomes can be significantly worse—more hospitalizations, more ICU admissions, more fatalities.
How It Spreads: Transmission Dynamics
Let’s talk mechanics. H1N1 is primarily spread through respiratory droplets—tiny particles expelled when an infected person coughs, sneezes, talks, or even breathes heavily. These droplets can travel short distances (usually less than six feet) and settle on mucous membranes of nearby people. It’s also possible to get infected by touching contaminated surfaces and then touching your eyes, nose, or mouth.
But beyond basic virology, real-world transmission is shaped by social behaviors and infrastructure. Crowded urban environments, mass gatherings, public transport, and schools can amplify spread dramatically. Think of how flu seems to explode in schools each winter—children are efficient viral vectors, often shedding virus before they even show symptoms.
And while seasonality affects transmission, it’s not just about cold weather. Colder air means drier indoor environments, which may help viral particles remain airborne longer. Plus, people spend more time indoors, increasing contact rates.
There’s also the role of asymptomatic or pre-symptomatic transmission. Some individuals can spread H1N1 before they feel sick—or without ever developing symptoms at all. This makes containment strategies like screening and isolation more difficult.
Epidemiology of Resistance: A New Layer of Complexity
Now let’s add drug resistance to the mix. Tracking the spread of resistant H1N1 isn’t just about virology anymore—it’s about molecular surveillance, international data sharing, and genomic sequencing.
Since 2009, oseltamivir-resistant strains of H1N1 have been detected in multiple countries. At times, these have emerged independently. At others, resistant strains have spread person-to-person within hospitals or communities.
So far, most resistant cases have remained isolated or sporadic, but some regional clusters have shown sustained transmission, particularly in immunocompromised patients or where antiviral usage is widespread. This pattern raises a troubling possibility: under the right conditions, resistant strains can propagate and even outcompete sensitive strains—especially if resistance mutations don’t significantly impair the virus’s fitness.
The upshot? Antiviral resistance isn’t just a lab curiosity—it’s an epidemiological reality, and one that’s likely to increase unless we improve stewardship and expand our antiviral arsenal.
Outbreak Case Studies: What We’ve Learned
Several outbreaks in the last decade have illustrated both the complexity and unpredictability of H1N1 epidemiology. Consider:
- India, 2015–2017: A surge in H1N1 infections led to thousands of deaths, particularly among younger adults. The virus appeared to have undergone minor antigenic changes, contributing to a mismatch with that year’s vaccine.
- United States, 2018–2019: While not a pandemic-level event, H1N1 was the dominant seasonal strain. Interestingly, cases of oseltamivir-resistant H1N1 were found in several states, particularly among patients with prior antiviral use.
- Japan, 2013: A small but significant cluster of oseltamivir-resistant H1N1 spread within a hospital setting, including to healthcare workers—a stark reminder of how quickly resistance can become a nosocomial concern.
Each of these events underscores a common lesson: H1N1’s behavior is shaped by human behavior, health systems, and ongoing viral evolution. Surveillance isn’t just important—it’s essential to staying one step ahead.
Clinical Manifestations
Let’s say you wake up one morning with a fever, dry cough, and sore muscles. Nothing too alarming at first—maybe it’s a cold, maybe it’s just fatigue. But by noon, your head is pounding, your whole body aches, and you’re alternating between chills and sweats. Is it just the seasonal flu? Or could it be H1N1? And more importantly—how do you tell when it’s becoming something worse?
H1N1 influenza can start like any other viral illness, which is both a blessing and a curse. On one hand, many cases are mild and self-limiting—especially in healthy individuals. On the other, its early presentation is so nonspecific that people often dismiss it, delay treatment, or mistake it for a benign cold. But beneath its common symptoms lies a potential for rapid escalation, especially when drug resistance limits treatment effectiveness.
The Typical Picture: Signs and Symptoms
At first glance, H1N1 influenza looks like your garden-variety flu. And that’s by design—after all, H1N1 is one of the culprits behind seasonal influenza. The virus generally has an incubation period of 1 to 4 days, meaning you can be infected and spreading the virus before symptoms even start.
When symptoms do appear, they often come on abruptly—a key feature that distinguishes flu from other respiratory viruses like the common cold. You might feel fine at breakfast and flattened by dinner.
Here’s what most patients report:
- Fever (often high and persistent)
- Chills and sweating
- Cough (typically dry, though it may become productive)
- Sore throat
- Headache
- Myalgias (muscle aches), often intense
- Fatigue or malaise
- Nasal congestion or runny nose
- Loss of appetite
Some patients, particularly children, may also experience gastrointestinal symptoms like nausea, vomiting, or diarrhea—less common in other flu types but more frequently seen with H1N1.
When It Gets Complicated: Red Flags for Severe Disease
Most people recover within a week or so. But not everyone is so lucky. For certain individuals, H1N1 can cause rapid deterioration, sometimes within 48 to 72 hours of symptom onset.
One of the most feared complications is viral pneumonia—a direct infection of the lungs by the influenza virus. This condition can cause:
- Shortness of breath
- Tachypnea (rapid breathing)
- Hypoxia (low oxygen saturation)
- Cyanosis (bluish discoloration of lips or fingers)
If not recognized and treated promptly, it can progress to acute respiratory distress syndrome (ARDS), a life-threatening condition where the lungs become severely inflamed and flooded with fluid. Patients may require mechanical ventilation and intensive care support.
Secondary bacterial pneumonia is another major concern, especially in older adults. After the initial viral assault, the immune system is weakened, allowing bacteria—most commonly Streptococcus pneumoniae, Staphylococcus aureus, or Haemophilus influenzae—to invade the lungs. This superinfection can cause a sudden relapse after a period of improvement.
Other serious but less common complications include:
- Myocarditis (inflammation of the heart muscle)
- Encephalitis (brain inflammation)
- Rhabdomyolysis (breakdown of muscle tissue, leading to kidney damage)
- Sepsis and multi-organ failure in critical cases
It’s worth emphasizing that these severe outcomes are more common in vulnerable populations—pregnant women, the immunocompromised, and those with preexisting respiratory or cardiovascular conditions.
What Does Resistance Change?
Now let’s add in the wrinkle of drug resistance. In uncomplicated cases, antiviral medications like oseltamivir can shorten illness duration and reduce symptom severity—if they’re started early (within 48 hours of symptom onset). But if the virus is resistant, those benefits diminish or disappear entirely.
This means clinicians are left with fewer options, and the infection has more time to progress unchecked. Resistance can also complicate decisions about post-exposure prophylaxis (preventive treatment after contact with an infected person), especially in settings like hospitals, nursing homes, or households with vulnerable individuals.
In essence, resistance can turn what might have been a moderate flu into a clinical tightrope walk, especially in patients already teetering due to comorbidities.
Special Considerations in Children and Pregnant Women
Children, especially those under five, may not present with classic flu symptoms. Instead, they might show signs like lethargy, irritability, poor feeding, or even seizures in severe cases. Parents should be on alert for rapid breathing, bluish skin tone, or unresponsiveness—all signs that urgent care is needed.
Pregnant women face a dual risk: not only are they more likely to develop complications from H1N1, but the infection can also affect the fetus, increasing the risk of preterm labor, fetal distress, and even miscarriage in severe cases.
It’s why both groups are prioritized for vaccination and early antiviral intervention, especially during flu season or outbreaks.
The Psychological Toll: Often Overlooked, Still Profound
One more note—less clinical, perhaps, but no less important. Severe H1N1 illness can have lasting effects not just on the body, but on the mind. Survivors who’ve endured ICU stays or prolonged recovery often report post-viral fatigue, depression, or even post-traumatic stress from the experience. This is particularly true for those who’ve been hospitalized during an outbreak when isolation protocols and fear of contagion add to the emotional strain.
As we learn more about the long-term impacts of viral infections (as with COVID-19), it’s becoming clear that flu recovery isn’t always straightforward—especially in those who faced resistance-related treatment failures.
Diagnosis
You walk into a clinic, coughing and feverish. It’s flu season. You’ve been in contact with a sick coworker. Maybe you’ve even tried some over-the-counter meds that haven’t helped. The doctor looks at you and suspects H1N1. But here’s the real question: how do they know for sure? And if antivirals are going to be used—especially in a world where drug resistance is increasingly on the radar—how do they know what treatment will actually work?
Diagnosing influenza, especially H1N1, sounds deceptively simple. But as any clinician will tell you, the reality is messier. The symptoms of flu overlap with dozens of other illnesses, and the tools we have for testing aren’t always perfect. Yet getting the diagnosis right is crucial—not just for your care, but for public health surveillance and outbreak control.
Clinical Diagnosis: A Useful Starting Point, But Far From Precise
In many settings—especially during peak flu season—clinicians often rely on clinical diagnosis. If you have a fever over 38°C (100.4°F), a dry cough, muscle aches, and fatigue, you’re likely to hear, “Sounds like the flu.” And in many cases, that’s a reasonable assumption.
But here’s the rub: clinical features alone can’t distinguish H1N1 from other influenza strains, COVID-19, RSV, or even bacterial infections. Especially in the early stages, these illnesses can look strikingly similar. This ambiguity becomes even more dangerous when the stakes are higher—say, in a pregnant woman or an elderly patient where timely treatment could mean the difference between recovery and ICU admission.
That’s where laboratory testing becomes vital. But even that’s not as clear-cut as it might seem.
Rapid Influenza Diagnostic Tests (RIDTs): Speed vs. Sensitivity
Let’s start with the tests you’re most likely to encounter in a typical clinic: Rapid Influenza Diagnostic Tests. These are the ones that promise results in 10 to 15 minutes, often using a nasal or throat swab. Sounds great, right?
The problem is that RIDTs are notoriously insensitive. They detect influenza antigens (parts of the virus), but they miss a significant portion of true infections, especially in adults. Some studies suggest that during H1N1 outbreaks, RIDTs may detect as few as 50–70% of actual cases.
In other words, a negative test result doesn’t rule out the flu—a point that can lead to missed diagnoses, delayed treatments, and incorrect assumptions about who is contagious.
Molecular Tests: The Gold Standard (with Caveats)
For greater accuracy, clinicians turn to RT-PCR (reverse transcription polymerase chain reaction). This test detects and amplifies the virus’s RNA, allowing not only for detection of influenza A but also for subtyping, so you can specifically identify H1N1.
RT-PCR is highly sensitive and specific. It’s the gold standard, especially in hospitals and reference labs. It can also detect low levels of virus before symptoms become severe—a crucial advantage for early treatment decisions or outbreak investigations.
But RT-PCR isn’t instant. It can take several hours to days, depending on the lab’s capacity. In fast-moving clinical scenarios—like in emergency departments or during a hospital outbreak—that time lag can be critical.
Also, PCR requires specialized equipment and trained staff. In rural or resource-limited settings, that’s not always available. So while PCR is excellent when you can get it, it’s not always accessible or timely.
Point-of-Care Molecular Tests: Bridging the Gap
Fortunately, in recent years, rapid molecular assays have begun to change the game. These are PCR-like tests that can be run near the patient—right in the clinic or emergency room—with results in 30 to 60 minutes.
Tests like Cepheid Xpert Flu/RSV or Abbott ID NOW provide higher accuracy than RIDTs and faster turnaround than lab-based PCR. They’ve become increasingly valuable tools during flu seasons and overlapping outbreaks with COVID-19.
Still, they come with cost and logistical hurdles, especially in high-volume settings or places without streamlined point-of-care workflows.
When Resistance Is Suspected: Diagnostic Blind Spots
Here’s where things get more challenging: how do you detect oseltamivir resistance in H1N1?
Unfortunately, standard flu tests won’t tell you anything about drug sensitivity. Even RT-PCR can confirm H1N1 infection without giving any clue about whether that virus is susceptible to antivirals.
To assess resistance, specialized molecular testing is required—often genotypic assays that look for known resistance mutations, like H275Y in the neuraminidase gene. But these tests aren’t routinely done, and they’re mostly used in public health labs, not standard clinical practice.
So what does that mean in real-world terms? It means many resistant infections are likely going undetected, particularly in patients who don’t improve after starting treatment. Resistance may only be suspected when a patient fails to respondto oseltamivir, prompting clinicians to either switch drugs (if possible) or send samples for further testing—which may take days.
This diagnostic blind spot is one of the reasons drug resistance in H1N1 is so concerning: we don’t always know it’s there until it’s already had clinical consequences.
Co-infections and Diagnostic Complexity
Another layer of complexity arises with co-infections. H1N1 can coexist with other respiratory pathogens—SARS-CoV-2, RSV, adenovirus, or bacteria like Streptococcus pneumoniae. These overlapping infections can obscure the clinical picture and impact treatment decisions.
Multiplex PCR panels that test for multiple viruses and bacteria at once have become valuable in such cases, especially in hospitals and during respiratory virus surges. But again, access and cost are barriers.
Clinical Judgement: Still the Front Line
Despite all this technology, one truth remains: clinical judgment is irreplaceable. Testing is essential, but so is understanding the patient’s history, risk factors, timing of symptoms, and context.
In suspected H1N1 cases—especially in high-risk groups or during outbreaks—empiric treatment may begin before test results are available, particularly if antivirals are to have maximum benefit. But that decision becomes thornier in the context of rising resistance, where we can no longer assume our go-to drug will be effective.
Treatment and Management
Imagine you’re a physician faced with a patient in the ER: high fever, labored breathing, PCR-confirmed H1N1. The patient is immunocompromised. You’re under pressure to act fast. You order oseltamivir—standard protocol. But what if the virus isn’t playing by the rules anymore? What if it doesn’t respond?
This is the daily tension at the heart of modern H1N1 treatment: our most trusted antivirals are becoming less reliable, and the margin for error is thin.
The Mainstay: Antiviral Therapies
Since the early 2000s, neuraminidase inhibitors have been the cornerstone of influenza treatment. These drugs—especially oseltamivir (Tamiflu), zanamivir (Relenza), and to a lesser extent peramivir (Rapivab)—work by inhibiting the neuraminidase enzyme on the virus’s surface, essentially trapping new viral particles inside the infected cell and halting further spread.
In uncomplicated flu, antivirals may reduce symptom duration by a day or so. But in severe or high-risk cases, early antiviral use can dramatically reduce hospitalization, complications, and mortality. The catch? Timing matters. These drugs are most effective when started within 48 hours of symptom onset.
Among the neuraminidase inhibitors, oseltamivir is the most widely used due to its oral formulation and ease of administration. Zanamivir, delivered via inhalation, is less convenient for many patients, especially those with asthma or COPD. Peramivir, an intravenous option, is reserved for hospitalized or critically ill patients who cannot take oral medications.
But here’s where the storm clouds gather: resistance is growing, especially to oseltamivir.
Resistance in Action: The Oseltamivir Problem
Let’s talk specifics. The most common resistance mutation in H1N1 is H275Y, a single amino acid change in the neuraminidase protein that dramatically reduces oseltamivir’s binding affinity. Viruses with this mutation can replicate and transmit just as efficiently—meaning they’re not just surviving, they’re thriving.
This isn’t merely theoretical. Clinical cases have emerged—some involving clusters of resistant infections, particularly in immunocompromised patients or those with prolonged antiviral exposure. Others have involved community-acquired resistance, where the patient had no prior antiviral use.
So, what happens when oseltamivir fails?
- If the patient can tolerate it and has no respiratory contraindications, zanamivir may still be effective. Resistance to zanamivir is less common, although it’s not nonexistent.
- Peramivir, administered IV, remains an option, but resistance mutations can reduce its efficacy too.
- Baloxavir marboxil (Xofluza), a newer antiviral that inhibits cap-dependent endonuclease (a different viral enzyme), is active against many oseltamivir-resistant strains. However, baloxavir has its own resistance issues, especially in pediatric patients and with single-point mutations like I38T.
In essence, we’re playing a game of antiviral whack-a-mole, where each new drug eventually provokes viral countermeasures.
Beyond Antivirals: Supportive and Critical Care
When antivirals alone aren’t enough—or when they’re delayed, ineffective, or resisted—supportive care becomes the main line of defense.
In mild to moderate cases, this might mean:
- Fluids and rest
- Antipyretics for fever and discomfort
- Monitoring for signs of deterioration (like shortness of breath, hypoxia, or confusion)
But in severe or hospitalized patients, care becomes more intensive:
- Oxygen therapy or noninvasive ventilation for respiratory distress
- ICU-level care, including mechanical ventilation for ARDS
- Broad-spectrum antibiotics if secondary bacterial pneumonia is suspected
- In extreme cases, extracorporeal membrane oxygenation (ECMO) may be necessary for refractory respiratory failure
Patients with preexisting conditions—like cardiovascular disease, obesity, diabetes, or pregnancy—require heightened vigilance, as these comorbidities can exacerbate influenza-related complications.
Immunocompromised Patients: A Unique Challenge
In patients with compromised immune systems—those undergoing chemotherapy, transplant recipients, or those with HIV—the story gets more complicated. These patients often experience prolonged viral shedding, giving the virus more time to mutate under drug pressure. They are also more prone to treatment failures and breakthrough resistance.
Sometimes, combination antiviral therapy is considered, though robust evidence is limited. Case reports have documented attempts to use dual neuraminidase inhibitors or neuraminidase inhibitors combined with baloxavir, but definitive clinical guidelines remain elusive.
Close monitoring and, when possible, genotypic testing for resistance are key in this population. It’s also where public health surveillance intersects with bedside medicine—because a resistant strain emerging in one immunocompromised host can, in theory, spark a broader outbreak.
Investigational Therapies: The Hopeful Edge
Given the cracks in our current antiviral arsenal, researchers are pursuing new treatment avenues. Some of the most promising include:
- Monoclonal antibodies targeting HA or NA proteins, designed to neutralize the virus directly. Several are in development, with some fast-tracked due to their potential for treating resistant strains.
- Broad-spectrum antivirals, like favipiravir and molnupiravir, which target viral RNA polymerase and may have utility in resistant cases.
- Host-targeted therapies, aimed at modulating the immune response (especially during cytokine storms) or inhibiting host proteins the virus depends on for replication.
Most of these remain in the experimental phase or under emergency use evaluation, but the future of influenza treatment may very well lie here—especially if resistance continues to rise.
The Real World: Management Isn’t Just About Medication
Let’s not forget: treating H1N1 also involves logistical and ethical decisions. During outbreaks, how do we prioritize limited antivirals? In the face of resistance, how do we manage public expectations, especially when treatment isn’t guaranteed to work?
And for healthcare workers, how do we protect ourselves and our colleagues while continuing to deliver care—particularly when personal protective equipment is scarce, or when institutional protocols are evolving?
Treatment doesn’t exist in a vacuum. It’s shaped by resource availability, public policy, health literacy, and even sociocultural beliefs about medication and vaccines. All of this must be factored into management strategies—especially as resistant strains create wider ripples across communities.
Prevention and Control
If you’ve followed H1N1’s story from its genetic quirks to its clinical mischief, you’re likely asking the most pragmatic question of all: how do we stop it? Or, if complete prevention isn’t possible, how do we keep it from spreading, evolving, and spiraling out of control—especially when our frontline antiviral treatments may no longer hold the line?
The answer, perhaps unsurprisingly, doesn’t lie in any one solution. It’s not a single drug, a single vaccine, or a single intervention. Instead, it’s a complex dance of science, strategy, behavior, and public infrastructure. And in the case of a virus as mutable and persistent as H1N1, prevention isn’t just a helpful bonus—it’s a critical necessity.
Let’s begin with vaccination, which remains our best defense, even in an imperfect world. Each year, flu vaccines are reformulated to match the strains scientists predict will be most prevalent. H1N1 has been included in this seasonal mix ever since its 2009 pandemic reintroduction. When the prediction is accurate—when the vaccine and circulating virus are well matched—the level of protection can be impressive. People are less likely to get sick, and if they do, the illness is often less severe. But even when the match is off, vaccination still offers benefits. It reduces the odds of hospitalization, serious complications, and transmission.
That said, the influenza vaccine is not magic. Its effectiveness is highly dependent on viral drift, production timelines, and the host’s immune response. A vaccine developed months before flu season begins might not perfectly align with the viral variants that end up dominating. Moreover, people with weakened immune systems, including the elderly and the chronically ill, may not respond as robustly to the vaccine as healthier individuals. And while this leads some to dismiss the shot’s value altogether, the broader truth is this: vaccination doesn’t just protect individuals. It slows the spread of infection within communities, reducing the overall disease burden. Even a modestly effective vaccine, when widely adopted, becomes a barrier to viral transmission.
This collective effect—known as herd immunity—is especially important for those who can’t protect themselves. Newborns, people with severe allergies to vaccine components, and the immunocompromised all depend on others being vaccinated. In this way, prevention isn’t just personal—it’s communal. Your flu shot, in a very real sense, shields someone else.
Still, vaccines are only part of the puzzle. The way people behave—individually and socially—can either enhance or undermine our best medical efforts. Hygiene practices like regular handwashing, covering coughs and sneezes, and staying home when sick may seem pedestrian, but they are incredibly effective. The challenge isn’t the science—it’s the consistency. During a flu season, even modest adherence to these behaviors can drastically reduce viral spread. But human nature often gets in the way. In cultures that reward “pushing through” illness, and workplaces where paid sick leave is a luxury, many people continue to go to work or school despite early symptoms. The result? Wider outbreaks. The virus thrives on our routines.
And let’s not ignore the built environment. Enclosed, poorly ventilated spaces—offices, schools, public transportation—create ideal conditions for airborne spread. Improvements in ventilation, even simple ones like increasing air exchange rates or adding HEPA filtration, are often overlooked but can make a real difference in transmission dynamics.
Then there’s the broader layer: public health infrastructure. Behind the scenes, a global surveillance network works year-round to track how influenza viruses—H1N1 included—are mutating, spreading, and behaving. The World Health Organization’s surveillance system collects samples from all over the globe, sequences them, and shares the data in real time. This surveillance is what allows us to reformulate vaccines, identify outbreaks early, and flag the emergence of drug-resistant strains.
When resistance does appear, public health teams move into containment mode. If an outbreak involves a resistant H1N1 variant, the response may include identifying and monitoring contacts, implementing isolation protocols, and distributing alternative antivirals from national stockpiles. These efforts work best when they’re fast, well-funded, and backed by clear communication. That last piece—communication—has proven particularly difficult in recent years. The rise of misinformation and vaccine hesitancy has made public health messaging more fragile than ever. If people don’t trust the message—or the messenger—no amount of scientific rigor can compel cooperation.
In certain high-risk environments, such as hospitals or nursing homes, the urgency is even greater. An outbreak in a long-term care facility can have devastating consequences. Staff vaccination, early detection protocols, and rapid prophylaxis are all essential tools in containing such events. Similarly, in schools and universities—settings that serve as natural amplifiers for flu transmission—proactive measures like student vaccination drives and policies that support sick days without penalty can be more impactful than any reactive response.
And then there’s the elephant in the room: what about resistant strains themselves? Can we prevent them? Not entirely. Resistance is, in part, the price of evolution. When a virus is exposed to a drug over and over again, natural selection favors mutations that help it survive. We can’t stop that process entirely, but we can influence its pace and its scope.
This is where antiviral stewardship comes into play. Prescribing antivirals thoughtfully—only when genuinely indicated, for the shortest necessary duration—reduces unnecessary selective pressure. In immunocompromised patients, where resistance tends to develop most readily, it’s especially important to tailor therapy carefully and monitor viral load when possible. And in the broader context, investing in the development of new antiviral agents and next-generation vaccines—including so-called “universal” flu vaccines that target stable parts of the virus—offers the best hope of future-proofing our defenses.
All of this points to a single conclusion: when it comes to H1N1, prevention isn’t passive. It’s active, multilayered, and adaptive. It involves everything from the vaccine you get at your local pharmacy to the genomic sequencing done halfway around the world. And as resistance looms larger, each layer becomes more important—not just to slow the spread, but to preserve the tools we still have.
Recent Developments (2025–2026)
By now, you might be wondering: with all this history, theory, and strategy—what’s actually happening now? How is H1N1 behaving in 2025 and 2026? Are we seeing more drug resistance? Are our vaccines keeping up? Has the virus thrown us any new curveballs? And most importantly—how is the global health system responding?
In many ways, the past two years have been an inflection point in our relationship with H1N1. Not because the virus has transformed into something radically different—but because the ecosystem around it has shifted. Our diagnostic tools are faster, more decentralized, and in some places even automated. Surveillance networks are more integrated, connecting hospitals, research centers, and international agencies in ways that would have seemed like science fiction a decade ago. And yet, despite these advances, the virus remains one step ahead in key areas—especially when it comes to resistance.
Let’s start with what we’ve learned from recent outbreaks. In early 2025, a cluster of severe H1N1 cases emerged in Buenos Aires during the Southern Hemisphere flu season. At first, there was nothing unusual about the presentation—typical flu symptoms progressing in some cases to pneumonia. But what set this cluster apart was its resistance profile. Samples sent to the national virology institute showed that several patients were infected with H1N1 strains carrying the H275Y mutation, long associated with oseltamivir resistance. What made this concerning wasn’t just the mutation itself, but its transmissibility. Unlike previous outbreaks where resistance appeared sporadically or only in heavily treated immunocompromised patients, this cluster involved healthy adults who had not received antivirals. In other words, the resistant virus was now circulating freely in the community.
This wasn’t an isolated incident. By mid-2025, a parallel event occurred in parts of Eastern Europe, particularly in Romania and Bulgaria. Again, resistant H1N1 strains appeared to be spreading among the general population. Molecular analysis revealed minor differences in the genetic background of the viruses compared to those seen in South America, suggesting that resistance was emerging independently in multiple regions—a sign of growing selective pressure on the virus globally.
Fortunately, these resistant strains have not yet demonstrated increased severity. Most cases remained within the spectrum of typical influenza. But that’s a cold comfort when treatment options narrow. Several hospitals began shifting their protocols to emphasize zanamivir and baloxavir, where available, and prioritizing molecular testing for hospitalized patients to identify resistance earlier.
Diagnostics have evolved in parallel. In 2025, the rollout of next-generation point-of-care molecular panels became widespread in many countries, especially those that previously depended on slower, centralized PCR testing. These compact systems—often the size of a small printer—can test for dozens of respiratory pathogens simultaneously, including markers of antiviral resistance. While not yet universal, their increasing presence in urban hospitals and even some large clinics is transforming the speed of decision-making. A patient who once waited 48 hours for a definitive result can now be diagnosed—and started on the appropriate treatment—in under an hour.
On the vaccine front, 2025 marked an important turning point. That year’s formulation, updated with newly circulating H1N1 sequences, produced an unexpectedly strong match in both hemispheres. The result was a significant reduction in hospitalizations compared to the previous three seasons. Researchers credited the match to a better integration of real-time sequencing data into the vaccine selection process, made possible by AI-assisted epidemiological forecasting models. For the first time, computational models helped virologists adjust vaccine strain recommendations just weeks before production deadlines, allowing greater responsiveness to late-emerging variants.
And while current flu vaccines are still strain-specific and seasonal, work on universal influenza vaccines has taken major strides in the last 18 months. Several candidates targeting the HA stalk (a more conserved portion of the virus than the head region that mutates frequently) have entered phase II and III trials. These vaccines aim to offer longer-lasting protection and cross-reactivity against multiple subtypes, including H1N1 variants that may be resistant to standard treatments.
But perhaps the most quietly significant development is the changing mindset among public health leaders. The H1N1 outbreaks of 2025–2026 haven’t triggered widespread panic—not because the virus isn’t serious, but because the systems for early detection, coordinated response, and informed risk communication are finally beginning to catch up with the pace of viral evolution. National health agencies now routinely release not just weekly flu incidence reports, but genomic resistance updates. Public communication strategies have shifted from paternalistic directives to transparent, dialogue-driven messaging—a subtle change, but one that builds trust and improves compliance.
Still, the future is not without its uncertainties. Resistance to zanamivir is slowly increasing in isolated pockets, particularly among patients with underlying lung disease. Baloxavir, while still effective against most circulating strains, has already shown signs of vulnerability in pediatric populations. And globally, access remains uneven. In many parts of sub-Saharan Africa and Southeast Asia, diagnostic and antiviral availability lag far behind, making containment of resistant strains more difficult.
There’s also a question looming in the background: will a new antigenic shift—an abrupt reassortment of influenza genes—bring a novel H1N1 variant capable of triggering another pandemic? So far, surveillance suggests no immediate threat. But history reminds us that influenza’s greatest trick is its unpredictability. All the tools in the world can’t prevent the emergence of something truly novel. What they can do—what they must do—is buy us time.
One thing is clear: the story of H1N1 isn’t static. It’s a living narrative, constantly shaped by science, policy, and biology. Resistance is real, but so is our capacity to adapt. And if we stay engaged—at the bedside, in the lab, in the public square—we may yet keep this persistent virus from regaining the upper hand.
FAQ — H1N1 Influenza and Drug Resistance
1. What is H1N1 influenza, and how is it different from other flu viruses?
H1N1 is a subtype of influenza A, defined by its surface proteins—hemagglutinin type 1 (H1) and neuraminidase type 1 (N1). While it behaves similarly to other flu viruses, it has a unique genetic history and has caused two major pandemics, in 1918 and 2009. Today, it circulates as one of the standard seasonal flu strains.
2. How serious is H1N1 infection?
Most cases are mild and self-limiting, especially in healthy individuals. However, H1N1 can cause severe illness or death in vulnerable populations, including children, the elderly, pregnant individuals, and those with chronic medical conditions. Complications like viral pneumonia, ARDS, and secondary bacterial infections are the main drivers of serious outcomes.
3. How does drug resistance in H1N1 happen?
Drug resistance arises when mutations—such as the H275Y mutation in the neuraminidase gene—alter the virus in a way that reduces the effectiveness of antiviral medications. These mutations often develop under selective pressure from widespread or prolonged antiviral use.
4. Are antivirals still effective against H1N1?
Yes, but with caveats. Oseltamivir remains useful for many cases, but resistant strains are on the rise. Alternatives like zanamivir, peramivir, and baloxavir offer other treatment options, though they too face resistance concerns. Early diagnosis and resistance monitoring are key to using these treatments effectively.
5. Can the flu vaccine protect me from H1N1?
Yes. Annual flu vaccines typically include an H1N1 component and offer protection, especially against severe illness. Even if the vaccine doesn’t perfectly match the circulating strain, it still reduces the risk of hospitalization and death.
6. How can I tell if I have H1N1 versus another flu or virus?
Only laboratory testing can definitively identify H1N1. Rapid tests can give quick answers, but PCR-based molecular tests are the most accurate. Clinical symptoms alone aren’t specific enough to differentiate H1N1 from other respiratory infections.
7. How is resistance tracked and diagnosed?
Genetic sequencing and specialized molecular assays can detect known resistance mutations, but these tests are not widely available in routine clinical settings. Most resistance detection currently occurs through public health surveillance rather than point-of-care diagnostics.
8. What’s being done to address drug-resistant H1N1?
Efforts include antiviral stewardship (to slow resistance), development of new antivirals and monoclonal antibodies, global genomic surveillance, and next-generation vaccine research—including universal flu vaccines targeting conserved viral components.
9. Is H1N1 likely to cause another pandemic?
Not in its current form. H1N1 is now a seasonal virus. However, influenza A viruses are prone to antigenic shift—a genetic reassortment that could produce a novel, pandemic-capable strain. Vigilant surveillance is essential to catch such shifts early.
10. How can individuals help prevent the spread of H1N1?
Get vaccinated annually, practice good hygiene, stay home when sick, and follow public health guidance during flu season. Your actions reduce both personal risk and the risk to others, especially those who are medically vulnerable.
Conclusion: Staying Ahead of the Curve
H1N1 is no longer the unfamiliar threat it once was. It’s woven into the fabric of seasonal flu cycles, quietly circulating each year, changing just enough to evade some of our defenses but not so much that it escapes our attention. Yet even in its familiarity, H1N1 still commands our respect—because what it lacks in drama, it makes up for in resilience.
This virus has taught us many things over the past century. It has shown us how quickly influenza can move through populations, how devastating a poorly understood strain can be, and how essential speed, coordination, and transparency are in both clinical and public health responses. In more recent years, it has underscored the fragility of our antiviral toolbox. As resistance emerges—not just in isolated cases but in transmissible clusters—we’re reminded that treatment is never static. A drug that works today may not work tomorrow.
But there is progress. Advances in diagnostics have made early and precise identification more feasible than ever before. Surveillance networks have matured, capable of tracking viral evolution in near-real time. Vaccination technology is pushing toward a more universal solution—one that might finally outpace the virus’s capacity to escape. These advances are deeply intertwined with the lessons learned from other respiratory pandemics, including our continuing experience with COVID-19, where rapid diagnostics and real-time genomic surveillance became cornerstones of response strategy.
Prevention, in this context, becomes more than a public health slogan. It becomes a practice—personal, clinical, and political. It means vaccinating not just for yourself, but for your community. It means prescribing antivirals with care, not abandon. It means recognizing that fighting flu isn’t just about reacting to outbreaks—it’s about building the systems, trust, and resilience to prevent those outbreaks from ever gaining ground.
As we look ahead, H1N1 will remain a presence—sometimes quiet, sometimes surging—but never inert. Whether it stays manageable or becomes a renewed crisis will depend not just on what the virus does next, but on what we do next.
And perhaps that’s the ultimate takeaway: the story of H1N1 is not only virological. It’s human. It’s about choices, preparation, and the shared effort to stay one step ahead in a world where pathogens don’t wait.