Marburg Virus: Understanding the Deadly Hemorrhagic Fever
Introduction
How did one of the most lethal viruses known to humanity slip into the scientific radar almost by accident? The story of the Marburg virus begins not in the depths of an African rainforest, but in the laboratories of Europe—an unsettling reminder that emerging infectious diseases can travel great distances before detection.
Brief History and Discovery of Marburg Virus
The year was 1967. In what was then West Germany and Yugoslavia, laboratory workers suddenly began falling ill with a mysterious and terrifying illness. It started like a common flu—fever, malaise, and muscle aches—but quickly spiraled into something far more alarming. Hemorrhaging, organ failure, and, in many cases, death followed. The source? A shipment of African green monkeys imported from Uganda, used in the production of polio vaccines.
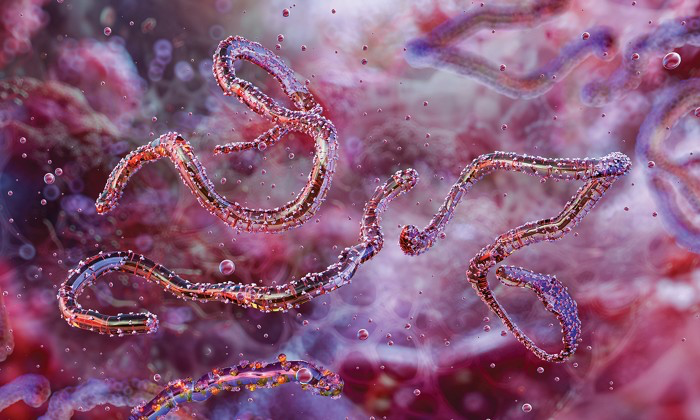
This incident led to the discovery of the Marburg virus, named after the German town where the first and most prominent outbreak occurred. Unlike many viral threats that quietly simmer in remote villages before making headlines, Marburg stormed onto the global stage through modern science itself—a cruel irony for a disease that we still struggle to understand and control.
Since then, Marburg has remained relatively rare, but its impact is always outsized. Each outbreak brings renewed urgency—and often panic—because of its extremely high mortality rate and the lack of a specific cure.
Comparison with Other Filoviruses Like Ebola
When most people hear “hemorrhagic fever,” they think of Ebola, and for good reason. Ebola has become a grim household name after several large and deadly outbreaks, especially in West Africa. But Marburg, often referred to as its “cousin,” is no less dangerous.
So how do the two compare?
Both Marburg and Ebola viruses belong to the Filoviridae family, and they share many biological and clinical features. They both cause severe hemorrhagic fevers, have similar routes of transmission (primarily through contact with infected bodily fluids), and are capable of rapid, devastating spread in healthcare and community settings.
But while Ebola has been the subject of more intensive public attention and research—largely due to its higher number of outbreaks and affected regions—Marburg is the quieter, equally deadly sibling. Some strains of Marburg virus have had case fatality rates exceeding 80%, which puts it in the same league, if not higher, than most Ebola strains.
Another key difference lies in the epidemiological footprint. Ebola has dominated in West and Central Africa, whereas Marburg outbreaks have primarily occurred in East and Central Africa, particularly in Uganda, Angola, and the Democratic Republic of the Congo. But that map is changing, as recent cases suggest.
Why does Marburg get less attention? Possibly because it has, until now, occurred in smaller outbreaks. But the potential for larger-scale epidemics is very real. The virus’s ability to spread in healthcare settings, its often-delayed recognition, and the absence of licensed treatments make it a public health threat that deserves far more spotlight.
In the next section, we’ll explore where Marburg virus tends to emerge, how it spreads, and what we’ve learned from its epidemiological patterns.
Epidemiology
If Marburg virus is so deadly, why don’t we hear about it more often? Where does it hide between outbreaks? And what makes it suddenly spill over into human populations? These are the kinds of epidemiological mysteries that scientists and public health experts have been trying to unravel for decades.
Understanding the “when, where, and how” of Marburg outbreaks is essential for stopping them before they spiral out of control. But the virus is elusive—its reservoir lies hidden, its spread unpredictable, and its outbreaks often explosive.
Geographic Distribution and Recent Outbreaks
Marburg virus is mostly a problem in sub-Saharan Africa, but its reach has varied over the years. Historically, outbreaks have occurred in Uganda, Kenya, Angola, the Democratic Republic of the Congo, and more recently, Equatorial Guinea and Ghana. These outbreaks are typically localized, but they can be extremely deadly, with case fatality rates ranging from 24% to over 80%.
The first recognized outbreak in Angola in 2004–2005 was a wake-up call. With over 250 reported cases and more than 200 deaths, it became the deadliest Marburg outbreak to date. Since then, sporadic cases have continued to emerge, particularly in Uganda’s remote cave systems and mining regions.
But what has changed recently? Why is Marburg making headlines again?
In 2025, new clusters of Marburg cases emerged in Rwanda and Equatorial Guinea, triggering global concern. These weren’t just isolated incidents—there were signs of community transmission, healthcare worker infections, and alarming delays in diagnosis. In Equatorial Guinea, for instance, delayed reporting led to potential cross-border exposure, raising red flags across West and Central Africa.
What makes these outbreaks even more concerning is that some regions had no previous history of Marburg virus activity. Could this indicate an expanded range for the virus? Or are we simply detecting cases now that would have gone unnoticed in the past? Either way, the trend is worrying.
Transmission Dynamics and Natural Reservoirs
Where does the Marburg virus come from? This is one of the most important—and perplexing—questions in its epidemiology.
The natural reservoir of the Marburg virus is believed to be the Egyptian fruit bat (Rousettus aegyptiacus). These bats are common throughout Africa and often roost in caves or abandoned mines—locations that humans sometimes enter for tourism, religious purposes, or mineral extraction.
So, how does the jump from bat to human occur?
Most likely, through direct contact with bat droppings, urine, or saliva, especially in enclosed spaces like caves where the virus can accumulate in the air or on surfaces. There have also been reports of people becoming infected after handling bat carcasses or consuming bushmeat—practices that are culturally embedded in some communities and difficult to change without strong public health education.
Once the virus infects a human, it becomes highly contagious. Person-to-person transmission occurs through direct contact with blood, secretions, or other bodily fluids of infected individuals. It can also spread through contaminated surfaces, particularly in healthcare settings where protective measures may be limited or inconsistent.
One of the more chilling aspects of Marburg transmission is what experts call “nosocomial amplification.” In under-resourced hospitals or clinics, the virus can spread like wildfire due to the reuse of needles, insufficient disinfection, or lack of personal protective equipment (PPE). In past outbreaks, healthcare workers have often been among the first—and worst—affected.
Interestingly, similar patterns of zoonotic spillover and healthcare-associated transmission have been observed with other high-risk viruses, such as the Nipah virus—a disease with its own complex interplay between bats, humans, and health infrastructure.
This raises another critical question: Can Marburg go global?
In theory, yes. While its primary reservoir and most outbreaks are based in Africa, international air travel means that an infected individual could carry the virus across continents before symptoms begin. Fortunately, Marburg’s rapid progression and high lethality have so far limited long-distance transmission chains. But as seen with Ebola, all it takes is one uncontained cluster to spark a full-scale public health emergency.
Pathogenesis
How does something as small as a virus—barely a wisp of genetic material wrapped in protein—cause such catastrophic damage in the human body? What is it about the Marburg virus that leads to bleeding from the eyes, collapse of internal organs, and death in a matter of days?
To understand the sheer destructiveness of Marburg virus disease, we need to look at the microscopic battlefield where the virus does its work: our cells, our immune systems, and ultimately, the very structure of our tissues.
Viral Structure and Replication
At its core, the Marburg virus is both simple and sinister. It belongs to the Filoviridae family, named for the filamentous, thread-like shape of its viral particles—some of which can reach up to 14,000 nanometers in length, though only 80 nanometers wide. Picture a tiny snake, curling and twisting its way into your cells.
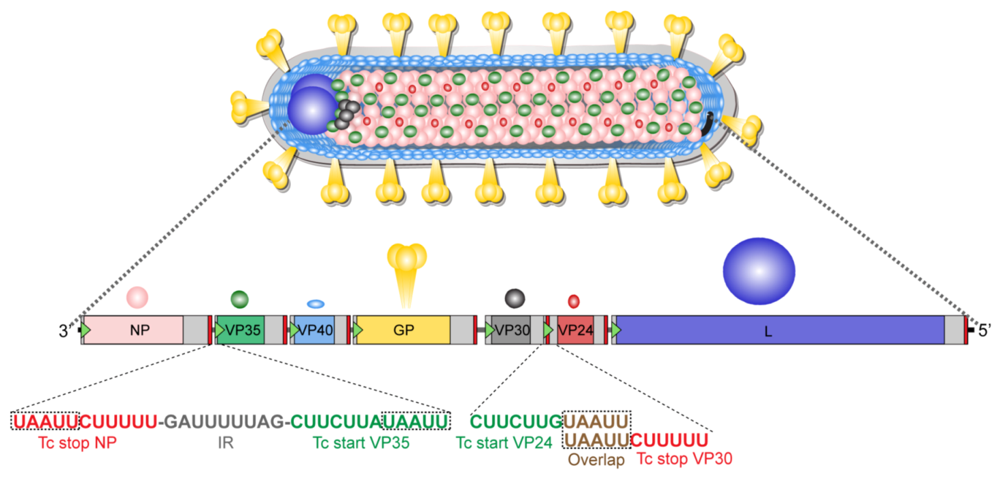
Its genetic material is composed of single-stranded, negative-sense RNA—not capable of doing much on its own, but once it gets inside a host cell, everything changes.
The virus targets cells that are rich in replication machinery: especially macrophages, dendritic cells, and endothelial cells. Once inside, it uses its own proteins to hijack the host’s cellular machinery, converting the cell into a virus factory. Thousands of new viral particles are produced and bud from the cell surface, spreading to infect nearby cells. This process continues relentlessly.
One of the most insidious aspects of Marburg’s replication is its stealth. During the early stages, the virus actually suppresses the host’s innate immune response, essentially blinding the body’s early-warning systems. By the time the immune system begins to react, the virus has already set up camp across multiple organ systems.
Impact on the Human Body and Immune Response
What happens once the Marburg virus has gained a foothold in the body?
The answer is: everything starts to break down.
The virus primarily targets immune cells and endothelial cells—the cells that line the interior of blood vessels. When these cells are damaged, vascular integrity collapses. In other words, the body starts to leak—literally. Blood seeps into tissues. Capillaries rupture. Internal bleeding begins. This is the basis for the term “hemorrhagic fever.”
But that’s just one piece of the puzzle. The immune response, once it finally activates, goes into overdrive, triggering what’s known as a cytokine storm. This massive release of inflammatory signals doesn’t just fight the virus—it inadvertently harms the host. Tissues become inflamed, organ function deteriorates, and multi-organ failure sets in.
Meanwhile, the liver is especially vulnerable. It becomes inflamed and begins to fail, worsening the already precarious coagulation problems. The kidneys may shut down. The spleen swells. Even the central nervous system can become involved, leading to confusion, seizures, or coma.
Why does Marburg kill some people and spare others? That’s still an open question. Some studies suggest that early viral load, the strength of an individual’s immune response, and genetic factors all play a role. Survivors tend to mount a faster and more regulated immune response, while fatal cases often show uncontrolled viral replication and widespread tissue destruction.
Another mystery? Why do some people survive with mild symptoms while others deteriorate rapidly? Are there unknown cofactors—perhaps related to nutrition, coinfections, or underlying health conditions—that tip the scales? The research is ongoing.
The immune system’s response—whether explosive or subdued—can mean the difference between survival and collapse.
You may also be interested in our detailed exploration of “CBC and Cancer Detection”, which connects closely with this topic. That piece delves into how routine lab markers can offer early signals of systemic distress—insights increasingly relevant to viral infections like Marburg.
What’s clear is that Marburg doesn’t just kill—it devastates, and often does so quickly. In many cases, death occurs within 8 to 9 days of symptom onset, often due to shock or severe blood loss.
Clinical Features
What does it feel like to be infected with the Marburg virus? Can you tell the difference between this and a common flu in the early stages? And how does a mild fever spiral into catastrophic organ failure within days?
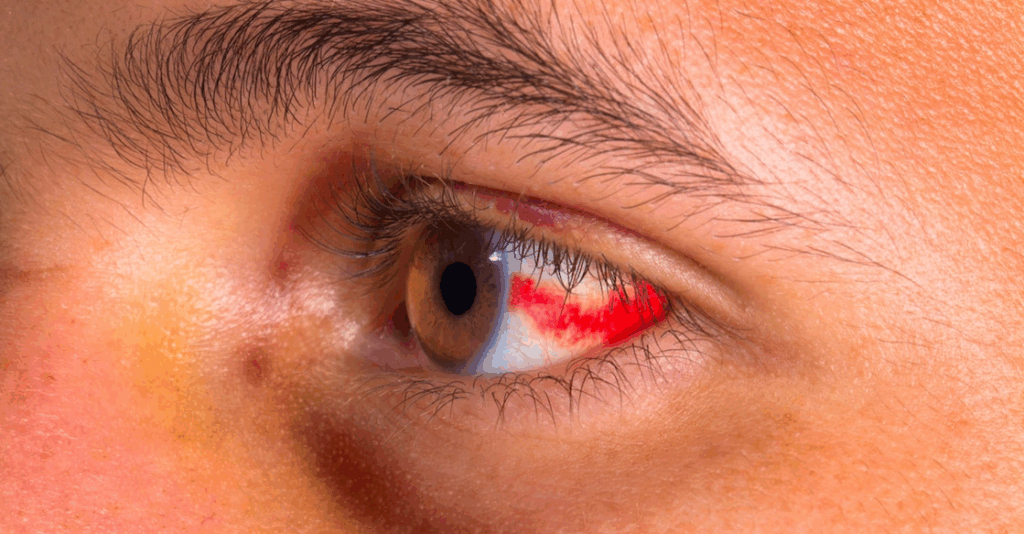
Recognizing the clinical course of Marburg virus disease is critical—not just for doctors, but for public health officials and anyone at risk. The virus hides in plain sight at first, but it quickly reveals its true nature. And by then, time is already running out.
Incubation Period and Symptom Progression
The incubation period for the Marburg virus generally ranges from 2 to 21 days, with most cases becoming symptomatic around 5 to 10 days after exposure. This silent phase can be especially dangerous because individuals may travel, work, or interact with others during this time, unknowingly spreading the virus.
When symptoms do appear, they start subtly, almost deceptively so. The early phase mirrors a variety of other febrile illnesses, which is part of why Marburg is so challenging to detect promptly. As the disease progresses, however, the symptoms escalate dramatically in severity and specificity.
By day 3 to 5 of illness, the clinical presentation becomes more severe and can include:
- Severe watery diarrhea
Often profuse and persistent, this diarrhea may continue for several days and can lead to severe dehydration, electrolyte imbalance, and physical exhaustion. It is one of the hallmark signs of Marburg in the acute phase and contributes significantly to patient deterioration. - Abdominal pain
Patients often report sharp, cramping pain across the abdomen. This discomfort is not just a side effect of the diarrhea, but may also result from inflammation of internal organs, such as the liver, spleen, or pancreas. - Nausea and vomiting
These symptoms further complicate fluid management and nutrition. Vomiting may contain blood in more advanced cases, indicating gastrointestinal bleeding, which is a serious prognostic sign. - Chest pain and sore throat
Chest pain may suggest pleuritis (inflammation of the lining of the lungs) or muscular involvement, while sore throat may be a result of lymphoid tissue inflammation or ulcerations in the oropharynx, further impairing swallowing and increasing discomfort. - Extreme fatigue
More than just tiredness, this exhaustion is debilitating, often rendering patients unable to stand or perform basic activities. The fatigue stems from both systemic inflammation and the body’s immense effort to fight off the infection.
As the disease continues into its later stages—usually after day 5 to 7—the signs of systemic and vascular failure begin to dominate the clinical picture:
- Bleeding from gums, nose, or gastrointestinal tract
This results from a breakdown of the vascular endothelium and coagulation pathways. Hemorrhages may be mild at first but can rapidly worsen, indicating widespread blood vessel damage. - Bruising and petechiae (tiny red or purple spots)
These are signs of capillary leakage and platelet dysfunction. Petechiae often appear on the trunk and limbs, while larger bruises may form spontaneously or with minimal trauma. - Hematemesis (vomiting blood) or melena (black, tarry stools)
Both are signs of internal bleeding, particularly in the upper gastrointestinal tract. These symptoms are very serious and often precede shock or death if not aggressively managed. - Neurological symptoms: confusion, irritability, agitation, or coma
These may be signs of brain inflammation (encephalopathy) or result from multi-organ failure affecting the brain’s function. Involvement of the central nervous system is a dire sign and usually associated with a poor prognosis.
Together, these symptoms paint a picture of a disease that moves swiftly from vague to vicious—one that begins like the flu and ends, in many cases, with uncontrolled bleeding and systemic collapse.
Severity and Mortality Rates
So, how bad does it get?
In some outbreaks, the case fatality rate has been recorded as high as 88%. In others, it’s lower—closer to 24–50%—but still alarmingly high. The wide range depends on several factors:
- The strain of Marburg virus
- The speed and quality of medical care
- Patient age and comorbidities
- Timing of supportive treatment
In general, death occurs most often between days 8 and 9, typically from massive blood loss, shock, or multi-organ failure. Survivors, on the other hand, usually begin to improve by the end of the second week—if they can hold on that long.
Interestingly, recovery isn’t always the end of the story. Some survivors report prolonged fatigue, vision problems, or hearing loss—what scientists refer to as post-viral sequelae. Like Ebola, Marburg may also persist in so-called “immune-privileged” sites, such as the testes or the eye, potentially reactivating or even transmitting to others long after the initial illness ends.
So the real question is: How do you distinguish Marburg virus from other diseases in its early stages, when symptoms are so vague? That’s where diagnostics come in—which we’ll explore in the next section.
Diagnosis
When someone presents with fever, fatigue, and diarrhea in a tropical region—how can you tell it’s not malaria or typhoid? Could it be Marburg virus? And how do you confirm that suspicion in time to save lives and prevent further spread?
Diagnosing Marburg virus disease (MVD) is a critical but challenging task. It requires not just a clinical eye, but also access to specialized testing—something not always available in outbreak settings. Rapid and accurate identification is vital for both patient management and epidemic control.
Laboratory Tests and Imaging Studies
RT-PCR (Reverse Transcription Polymerase Chain Reaction)
This is the primary and most reliable method for detecting Marburg virus, especially in the acute phase. It works by amplifying viral RNA in the patient’s blood, allowing detection within a few hours. RT-PCR is both sensitive and specific, but it requires well-equipped laboratories and trained technicians—often unavailable in rural areas where outbreaks begin.
ELISA (Enzyme-Linked Immunosorbent Assay)
ELISA tests can detect either IgM antibodies, which indicate recent infection, or viral antigens, showing the presence of active virus. These tests are useful for early diagnosis and retrospective analysis but may not pick up very early infections before the immune response kicks in.
Virus Isolation in Cell Culture
This method involves growing the virus from patient samples in a controlled laboratory setting. While definitive, it’s rarely used during active outbreaks due to its lengthy process and high biohazard risk. It’s mainly reserved for research or confirmation in specialized facilities.
Immunohistochemistry
Used for tissue analysis, particularly in post-mortem cases, this method visualizes the virus in infected organs using tagged antibodies. It’s informative for understanding the spread of the virus in the body but not suitable for rapid clinical diagnosis.
Next-Generation Sequencing (NGS)
NGS provides deep insight into the genetic makeup of the virus, helping track mutations and trace the source of outbreaks. It’s invaluable for epidemiological investigations but too slow and complex to serve as a frontline diagnostic tool.
Imaging Studies
Imaging like X-rays or CT scans offer little in the way of early diagnosis. By the time organ damage is visible, the disease is often far advanced. Imaging might show enlarged organs, fluid accumulation, or signs of bleeding, but none are specific to Marburg virus.
Differential Diagnoses
Malaria
Still the most common cause of fever in sub-Saharan Africa. Malaria can mimic early Marburg with chills and fatigue but typically lacks bleeding or rapid shock. Blood smears and rapid diagnostic tests are used to confirm or rule it out quickly.
Typhoid Fever
This bacterial infection causes prolonged fever and gastrointestinal issues but rarely results in hemorrhage unless severely advanced. Blood cultures or Widal tests are useful in distinguishing it from viral hemorrhagic fevers.
Dengue and Yellow Fever
These are viral illnesses transmitted by mosquitoes. Both can cause fever and bleeding in severe cases, which can overlap with Marburg, but their epidemiological context (urban mosquito exposure vs. bat contact) helps differentiate them. Rapid tests for dengue antigen or yellow fever serology can provide clarity.
Lassa Fever and Ebola Virus Disease
Close relatives of Marburg in terms of symptoms and transmission. Ebola and Marburg are especially hard to tell apart clinically, so lab tests are essential. Lassa may cause more hearing loss and neurological complications, while Ebola may have higher initial mortality.
Leptospirosis and Rickettsial Infections
These bacterial diseases can cause fever, liver dysfunction, and muscle aches, often mistaken for viral fevers. However, they respond well to antibiotics like doxycycline, making early diagnosis life-saving.
Septicemia and Meningitis
When patients present with confusion, coma, or high fevers, bacterial sepsis or meningitis must be considered. Unlike Marburg, these conditions often involve very high white blood cell counts and may be confirmed through blood or cerebrospinal fluid testing.
When a patient appears during or near an outbreak, and especially if they’ve had contact with bats or infected individuals, Marburg should be high on the list of suspects. The earlier it’s recognized and tested, the more chance there is to prevent an outbreak and begin effective containment.
Treatment
What do you do when there’s no cure? When the virus moves faster than most supply chains, and even well-equipped hospitals can only do so much? How do you care for a patient knowing that the virus they carry is one of the most lethal known to science?
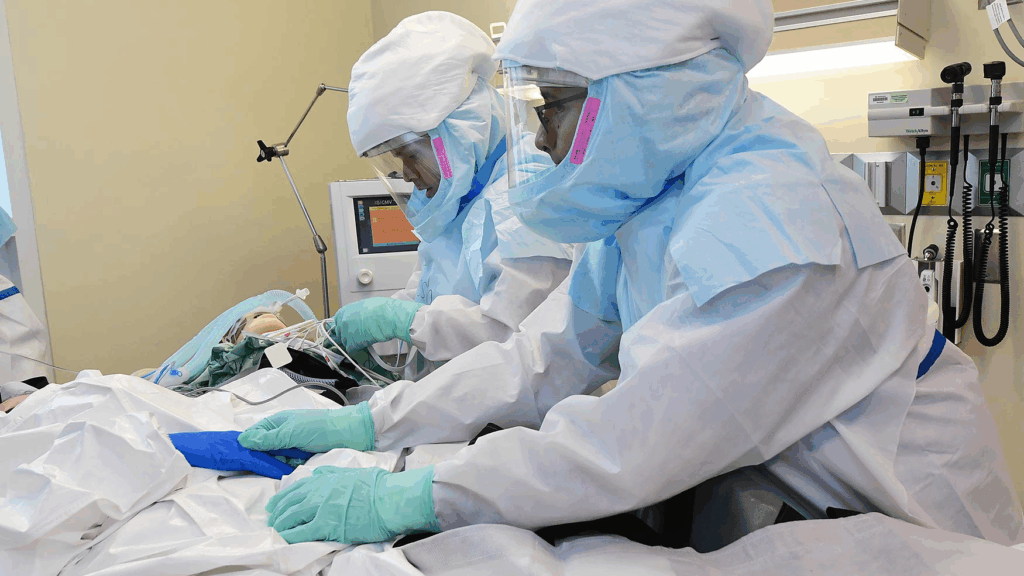
Treating Marburg virus disease (MVD) is a lesson in humility—and urgency. There are no licensed antiviral drugs or proven cures as of 2025, which means the focus remains on supportive care. But supportive doesn’t mean passive. In many cases, it’s the difference between life and death.
Supportive Care Measures
The core of Marburg treatment lies in aggressively managing symptoms and stabilizing vital functions. These interventions may sound basic, but when done well—and early—they dramatically improve survival.
Fluid and Electrolyte Management
One of the first and most important steps is to replace lost fluids. Patients with Marburg virus often experience severe diarrhea and vomiting, which leads to rapid dehydration and electrolyte imbalances. Without prompt correction, this can cause renal failure, arrhythmias, and shock.
Intravenous fluids (IVs) are essential. In high-resource settings, fluids are adjusted based on lab data. In lower-resource settings, oral rehydration salts may be used as a stopgap—but are rarely enough for critical cases.
Oxygen Therapy and Respiratory Support
Some patients develop respiratory distress or hypoxia (low blood oxygen). Supplemental oxygen can support vital organs and reduce the risk of cardiac arrest. In severe cases, mechanical ventilation may be needed, though this is often impractical in outbreak zones due to limited ICU resources and infection risk.
Hemodynamic Monitoring and Blood Pressure Support
As the infection progresses, many patients go into hypovolemic or septic shock. Constant monitoring of blood pressure, heart rate, and perfusion is necessary. When blood pressure drops dangerously, vasopressors (drugs that constrict blood vessels) may be used to maintain circulation.
Blood Products and Coagulation Support
Marburg virus disrupts clotting. Patients may bleed internally or externally. In some cases, doctors use blood transfusions, platelets, or plasma to support the coagulation system. This requires a safe, screened blood supply—a major challenge in outbreak zones.
Pain Management and Antipyretics
High fevers, muscle aches, abdominal pain, and headaches are common. While these symptoms may seem minor in the context of hemorrhagic fever, controlling them is important. It improves comfort, reduces stress on the body, and prevents further complications like seizures.
Nutritional Support
Because of nausea, vomiting, and overall weakness, many patients are unable to eat. Nutritional support, whether through oral feeding, supplements, or tube feeding, helps maintain body strength during the recovery phase. Malnourished patients tend to fare worse, so nutrition isn’t a luxury—it’s part of survival.
But even with the best supportive care, mortality can still be high. That’s why research into targeted therapies is ongoing—and hopeful.
Investigational Therapies and Vaccines
Are we getting closer to a cure—or at least a treatment? In the last decade, scientific momentum around Marburg has picked up, driven in part by lessons learned from Ebola. Several experimental therapies and vaccines are now in development or in early-stage deployment during outbreaks.
Antiviral Agents
A number of drugs are being tested for Marburg, including:
- Remdesivir, originally developed for Ebola and used during the COVID-19 pandemic, has shown modest effectiveness in animal models of Marburg, but data in humans is limited.
- Favipiravir, another RNA polymerase inhibitor, has been studied but with mixed results.
- Newer compounds targeting viral replication or immune modulation are in preclinical testing, some showing promise in monkeys and rodents.
The challenge? These drugs are not yet approved, and access during outbreaks is restricted to compassionate use or experimental protocols.
Monoclonal Antibodies
Much like the antibody therapies used for Ebola (e.g., Inmazeb, Ebanga), researchers are developing monoclonal antibodies specific to Marburg virus. These antibodies are designed to neutralize the virus directly and help the immune system clear it faster.
As of 2025, a few candidates have entered Phase I or II trials, but none are yet widely available. Nonetheless, in outbreak zones, they may be deployed under emergency protocols or for healthcare workers at high risk.
Vaccines
This is where the most progress has been made. Several Marburg vaccine candidates are in development, including:
- cAd3-Marburg, a chimpanzee adenovirus vector-based vaccine similar to the one used against Ebola, has shown good safety and immune response in Phase I trials.
- rVSV-Marburg, based on the vesicular stomatitis virus platform (used successfully in the Ebola vaccine Ervebo), is further along and may be deployed under ring vaccination protocols during outbreaks.
In 2026, limited trials began in Equatorial Guinea and Rwanda, targeting healthcare workers and close contacts during outbreaks. While not yet licensed, the momentum suggests that a viable Marburg vaccine may finally be within reach.
In the end, treating Marburg isn’t just about IV drips and fever reducers—it’s about buying time for the body to mount an immune response. Every small intervention—every drop of fluid, every calmed heartbeat—can make a difference.
But prevention is better than cure. And when there is no cure, prevention becomes everything.
Next, we’ll explore how Marburg is contained, what public health measures are used during outbreaks, and why personal protective equipment is only one part of a much larger picture.
Prevention and Control
When there’s no cure, no widely approved vaccine, and the virus can kill within days—how do you stop it? How do you protect a community, a country, or even a single hospital ward from something as ruthless as Marburg virus?
The answer lies in layers of protection—some technical, some logistical, and many deeply human. Prevention and control of Marburg virus outbreaks require a combination of public health coordination, personal protection, surveillance, and trust. And in many ways, it’s a race against time and fear.
Public Health Measures During Outbreaks
Stopping Marburg starts with early detection and swift response. A delay of even a few days in recognizing an outbreak can allow the virus to spread rapidly through families, hospitals, and entire communities.
Outbreak control doesn’t happen in isolation—it’s part of a larger public health landscape shaped by ecology, mobility, and trust.
Additionally, don’t miss our coverage of “Avian Influenza H5N1”, offering insights that complement this article. It explores how another zoonotic threat challenges the boundaries of containment, public messaging, and global cooperation.
Case Identification and Isolation
The first step is identifying suspected cases. This means training local health workers to recognize early signs and symptoms, especially in high-risk areas or during outbreaks. Once a case is identified, the person must be immediately isolated—not just for their own treatment, but to prevent further transmission.
But what does isolation actually involve?
It’s not just about moving someone into a separate room. It means dedicated care teams, separate sanitation, separate waste disposal, and strict no-contact protocols. In outbreak zones, entire treatment units are constructed using tents, tarps, and mobile facilities—with infection zones marked clearly and security posted to prevent breaches.
Contact Tracing
Every person who had close contact with an infected individual—whether it was a nurse, a family member, or a fellow bus passenger—needs to be identified and monitored for 21 days, the maximum incubation period. This is often a Herculean task in rural areas where recordkeeping is minimal and stigma is high.
Contact tracing isn’t just detective work—it’s an act of community engagement. Teams often have to build trust, explain why someone is being monitored, and counter local fears that health workers are spreading disease rather than preventing it.
Quarantine and Movement Restrictions
If contact tracing reveals widespread exposure, public health officials may impose quarantines or travel restrictions, especially between villages or across borders. While controversial, these steps can be essential in limiting virus spread. But they must be balanced with human rights and local cooperation—overreach can lead to resistance, which in turn, fuels the outbreak.
Safe Burial Practices
Traditional burial rites in many cultures involve washing and touching the deceased, which tragically makes funerals a major point of viral transmission. Marburg virus remains active in the body after death—sometimes for days.
Outbreak response teams must therefore conduct “safe and dignified burials”, where families are consulted, rituals are adapted, and bodies are handled by trained personnel in full protective gear. This process is as much about cultural sensitivity as it is about infection control.
Community Education
Misinformation spreads faster than viruses. During outbreaks, communities are often flooded with rumors—about who’s to blame, where the virus came from, or how to treat it.
Effective response depends on clear, consistent, and empathetic communication. Public health workers use radio broadcasts, posters, village meetings, and trusted community leaders to convey accurate messages about symptoms, transmission, and what to do when someone falls ill.
Personal Protective Equipment and Isolation Protocols
When dealing with a virus like Marburg, the gear healthcare workers wear isn’t just about protocol—it’s survival.
Personal Protective Equipment (PPE)
Full-body PPE is standard for anyone caring for a Marburg patient. This includes:
- Impermeable gowns or suits
Designed to prevent any exposure of the skin to infected fluids. - Gloves (double or triple layers)
To prevent viral entry through cuts or contact, especially during procedures. - N95 or higher-grade respirators
While Marburg is not airborne in the conventional sense, aerosols may occur during certain procedures like intubation or suctioning. - Face shields and goggles
To prevent splashes to the mucous membranes—an underappreciated route of infection.
But PPE is only effective when used correctly. One of the most dangerous moments in care is removal of contaminated gear, where a single slip can lead to infection. That’s why donning and doffing procedures are practiced repeatedly in training drills, often supervised by another staff member.
Decontamination and Environmental Control
Patient rooms, bedding, and waste must be treated as biohazardous. Surfaces are cleaned with strong disinfectants like bleach. Waste is burned or buried in secure pits. Equipment is either disposable or rigorously sterilized.
Even the airflow in treatment areas is considered: high-risk zones are often designed with negative pressure systems to contain any potential aerosols.
Health Worker Monitoring and Support
Healthcare workers are heroes—but they are also among the most vulnerable. During past outbreaks, infections among medical staff have not only increased the toll but have shattered public trust.
Protocols now include daily symptom checks, mental health support, and even post-exposure monitoring for workers in direct care roles. If an exposure is suspected, prophylactic or investigational treatments may be offered on a case-by-case basis.
In short, prevention and control of Marburg virus disease is not just a checklist of technical tasks. It’s a human-centered emergency response that blends science, logistics, psychology, and ethics.
And despite all this, the virus can still surprise us. Which is why we now turn to the most recent developments in Marburg outbreaks—and what they reveal about the virus’s evolving threat.
Recent Developments (2025–2026)
Has the Marburg virus changed? Or have we changed—our climate, our interactions with wildlife, our ability to detect outbreaks more quickly? What’s clear is this: the virus is no longer confined to its historical strongholds. In recent years, it’s reappeared in new places, under new circumstances, and with implications that stretch beyond national borders.
Let’s explore what the most recent outbreaks have taught us—not just about Marburg virus itself, but about the fragile systems we rely on to keep it contained.
Outbreaks in Rwanda and Equatorial Guinea
In early 2025, reports emerged from Rwanda of an unusual cluster of febrile illnesses with bleeding manifestations in a rural district bordering the Nyungwe Forest National Park. Initially dismissed as a possible flare-up of malaria or typhoid, it wasn’t until two healthcare workers fell seriously ill that samples were rushed to a regional reference lab. The diagnosis? Marburg virus disease—the first confirmed case in Rwanda’s history.
This event shocked regional health agencies. Rwanda had no known history of Marburg outbreaks, and yet here it was: a confirmed cluster with evidence of limited human-to-human transmission. The index case was eventually traced to a local man who had visited a forest cave inhabited by Egyptian fruit bats, long suspected to be the virus’s natural reservoir.
Just months later, another outbreak flared in Equatorial Guinea, this time in the coastal region of the Río Muni mainland. Unlike the previous isolated case reports in the country, this outbreak involved multiple transmission chains, including spread within a hospital, a prison facility, and even a border checkpoint. The outbreak resulted in 27 confirmed cases and 19 deaths, drawing swift international attention.
What made this outbreak different?
First, delayed recognition allowed the virus to move silently through two provinces before health authorities were alerted. Second, limited laboratory capacity meant that samples had to be flown to foreign labs for confirmation, adding days to the timeline. Third, the outbreak occurred in a politically sensitive region, complicating international collaboration and community engagement.
These events underscored several sobering truths:
- Marburg can emerge anywhere bats exist—not just where we’ve seen it before.
- Under-detection remains a major risk, especially in areas with poor health infrastructure.
- Political will, trust, and transparency are just as important as testing kits and isolation tents.
Research on Transmission from Bats to Humans
If we know that Rousettus aegyptiacus fruit bats are the likely reservoir of Marburg virus, why don’t we see more spillover events? And what causes some people to become infected while others walk through bat caves without incident?
These are precisely the kinds of questions scientists have been racing to answer, and 2025 brought new insights.
A multi-country study coordinated by the African Pathogen Surveillance Network (APSN) investigated the viral dynamics in bat populations across Uganda, Rwanda, Gabon, and Cameroon. Researchers found that viral shedding in bats appears to be seasonal, with peaks during birthing and weaning periods. During these times, juvenile bats are more susceptible to infection—and more likely to transmit the virus through saliva, urine, and feces.
Interestingly, many of the recent human index cases in Rwanda and Equatorial Guinea occurred shortly after peak bat reproductive cycles, strengthening the theory that timing matters. Human activities—such as cave tourism, bushmeat hunting, or crop harvesting near roosting sites—can inadvertently bring people into closer contact with bats during these high-risk windows.
Another key finding? Not all bat colonies carry the virus. Genetic sequencing revealed distinct Marburg virus variantscirculating in different bat populations—some more closely related to strains found in Angola or the DRC, others suggesting new evolutionary branches. This raises the possibility that the virus may be evolving faster than we thought, potentially adapting to new environments or hosts.
Perhaps most intriguingly, researchers identified low-level, asymptomatic infections in a handful of local villagers who regularly handle bats. Blood samples revealed antibodies to Marburg virus, suggesting past exposure without illness. Could this mean that mild or subclinical cases are more common than we think? If so, our current understanding of outbreak size—and immunity—might be incomplete.
In short, the Marburg virus is no longer simply a sporadic forest pathogen. It’s a virus on the move—shaped by ecology, travel, health infrastructure, and the ways we interact with nature.
In the final section, we’ll explore what this all means for the future: What will it take to stay ahead of Marburg? And how can the lessons of the past two years guide us toward better preparedness, resilience, and response?
FAQ: Marburg Virus Disease
1. What is the Marburg virus?
Marburg virus is a highly virulent pathogen from the Filoviridae family, the same group that includes Ebola. It causes a severe hemorrhagic fever in humans, often leading to multi-organ failure and death.
2. Where did the Marburg virus come from?
The virus was first identified in 1967 during outbreaks in Germany and Yugoslavia, linked to imported African green monkeys. However, its true reservoir appears to be Egyptian fruit bats, which are found across sub-Saharan Africa.
3. How is the Marburg virus transmitted?
It spreads through direct contact with the blood or bodily fluids of infected individuals, contaminated surfaces, or exposure to infected bats. There is no evidence of airborne transmission in casual settings, but certain medical procedures can generate infectious aerosols.
4. What are the symptoms of Marburg virus disease?
Symptoms begin like the flu—fever, chills, muscle pain—but rapidly worsen to include severe diarrhea, vomiting, internal and external bleeding, confusion, and shock. The disease can progress rapidly, with death often occurring within a week to ten days.
5. How is Marburg diagnosed?
Diagnosis requires laboratory testing, especially RT-PCR, ELISA, or antigen detection assays. These tests must be conducted in high-biosafety laboratories and are often centralized in outbreak regions.
6. Is there a cure or treatment for Marburg virus?
There is no specific antiviral treatment. Care is supportive, including fluid replacement, oxygen therapy, and organ support. Some experimental drugs and monoclonal antibodies are in development, and investigational use is possible in outbreak scenarios.
7. Are there vaccines for Marburg virus?
Several vaccine candidates are in development, such as rVSV-Marburg and cAd3-Marburg. Limited trials have begun in outbreak settings, but no vaccine has been fully licensed for widespread public use as of 2026.
8. How can Marburg virus outbreaks be prevented?
Prevention involves avoiding contact with bats, especially in caves or mines, and strict infection control practicesduring outbreaks. Public education, safe burial practices, and robust contact tracing are all crucial.
9. Can Marburg virus cause a global pandemic?
While Marburg virus is deadly, it spreads through direct contact, not airborne transmission. This limits its pandemic potential compared to respiratory viruses. However, delayed detection, global travel, and health system gaps mean that large regional outbreaks remain a serious risk.
10. What should you do if you suspect a Marburg infection?
Seek immediate medical attention, avoid contact with others, and report your symptoms to local health authorities, especially if you’ve had contact with wildlife or someone ill. Isolation and early diagnosis can save lives.
Conclusion
What makes Marburg virus so terrifying isn’t just its lethality—it’s the silence before the storm. The subtle beginning, the delayed recognition, the remote locations where health systems are thinly stretched. It’s a virus that exploits not just biology, but weaknesses in human systems.
The outbreaks of 2025 and 2026 showed that the threat is evolving. New regions are reporting cases. New variants may be emerging. But more importantly, new lessons are being learned—about how to detect the virus earlier, protect communities, and respond faster.
Moving forward, the key to outpacing Marburg lies in:
- Surveillance systems that are decentralized, fast, and trusted.
- Preparedness protocols that reach beyond capital cities into rural zones.
- Cross-border cooperation, since viruses don’t respect political boundaries.
- And crucially, investment in research—for diagnostics, treatments, and vaccines.
Marburg may not make headlines every day, but it should never be forgotten. Because the next outbreak isn’t a question of if, but when—and how ready we are when it arrives.